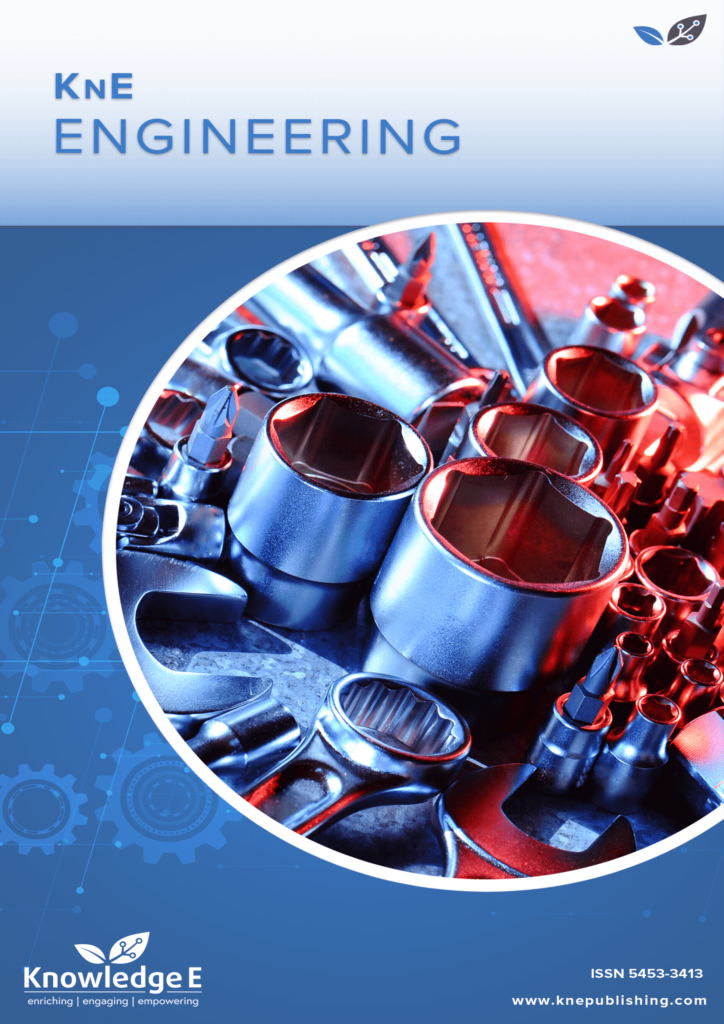
KnE Engineering
ISSN: 2518-6841
The latest conference proceedings on all fields of engineering.
Adaptation of Radiative Properties of the End Products of Fuels Combustion within the Temperature Range of 1,000…2,000 K
Published date: Jul 17 2018
Journal Title: KnE Engineering
Issue title: VII All-Russian Scientific and Practical Conference of Students, Graduate Students and Young Scientists (TIM'2018)
Pages: 185–192
Authors:
Abstract:
The accuracy of calculating heat exchange by radiation from high-temperature gas flow produced during natural fuels combustion to a large extent depends on the accuracy and status of data on thermophysical properties of gases and the value of the radiative heat flux. The main physical load is carried by the density of the intrinsic heat flux, but all the experimental data on gas mixtures radiation are given as a total emissivity of the components and the mixture in general. That is why this study determines the emissivity factor of carbon dioxide and water vapour as the main constituents of the products of industrial fuel combustion. Dependencies are developed based on reliable experimental data and allowed to perform emissivity factor calculations for the products of combustion. The accuracy of calculated approximation is determined for experimental data in the field of two factors: optical density of gas, and its temperature. The study results are recommended to be used
for developing heat exchange calculation programs.
Keywords: emissivity factor, radiation flux, temperature, carbon dioxide, water vapour
References:
[1] Telegin, А. C., Shvydky, V. S., and Yaroshenko, Yu. G. (2002). Heat-mass Transfer (2nd edition). Moscow: Akademkniga.
[2] Shvydky, V. S., Spirin, N. A., Ladygichev, M. G., et al. (1999). Elements of the Theory of Systems and Numerical Methods for Modeling the Processes of Heat and Mass Transfer. Moscow: Internet Engineering.
[3] Zobnin, B. F., Kazyayev, M. D., Kitaev, B. I., et al. (1982). Thermal Engineering Calculations of Metallurgical Furnaces. Moscow: Metallurgy.
[4] Blokh, A. G. (1984). Heat Exchange in the Furnaces of Steam Boilers. Leningrad: Energoatomizdat.
[5] Petuhova, B. S. and Shikova, V. K. (1987). Reference Book on Heat Exchangers, vol. 2; vol.1 (Russ.). Moscow: Energoatomizdat.
[6] Edwards, D. K., (1976). Thermal radiation measurements, in E. R. G. Eckert and R. J. Goldstein (eds.) Measurements in Heat Transfer, chapter 9. Washington, D. C.: Hemisphere.
[7] Gordon, Y. M. and Toropov, E. V. (2013). Scale-up problems of new alternative pyrometallurgical technologies, Energy and resource saving in heat and power engineering and social sphere (materials of the International scientific and technical conference of students, post-graduate students, scientists), pp. 5–12. Chelyabinsk:
Publishing Center of SUSU.
[8] Toropov, E. V. (2016). The systemically structured adaptation of heat transfer in boilers. Bulletin of South Ural State University, Series—Power Engineering, vol. 16, no. 1, pp. 19–23.
[9] Sparrow, E. M. and Sess, R. D. (1971). Heat Exchange By Radiation. Leningrad: Energy.
[10] Kutateladze, S. S. (1990). Heat Transfer and Hydrodynamic Resistance (reference book), Moscow: Energoatomizdat.
[11] Khrustalev, B. M., et al. (2007). Heat and Mass Transfer, in A. Nesenchuk (ed.), vol. 2, vol. 1. Minsk: Belarusian National University.
[12] Pomerantsev, V. V., Arefiev, K. M., Akhmedov, D. B. et al. (1986). Fundamentals of the Practical Theory of Combustion, in V. V. Pomerantseva (ed.). Leningrad: Energoatomizdat.
[13] Shvydky, M., Ladygichev, G., and Shavrin, V. S. (2001). Mathematical Methods of Thermophysics. Moscow: Mechanical Engineering.
[14] Blokh, A. G., Zhuravlev, Yu. A., and Ryzhkov, A. N., (1991). Radiation Heat Transfer (reference book). Energoatomizdat: Energoatomizdat.