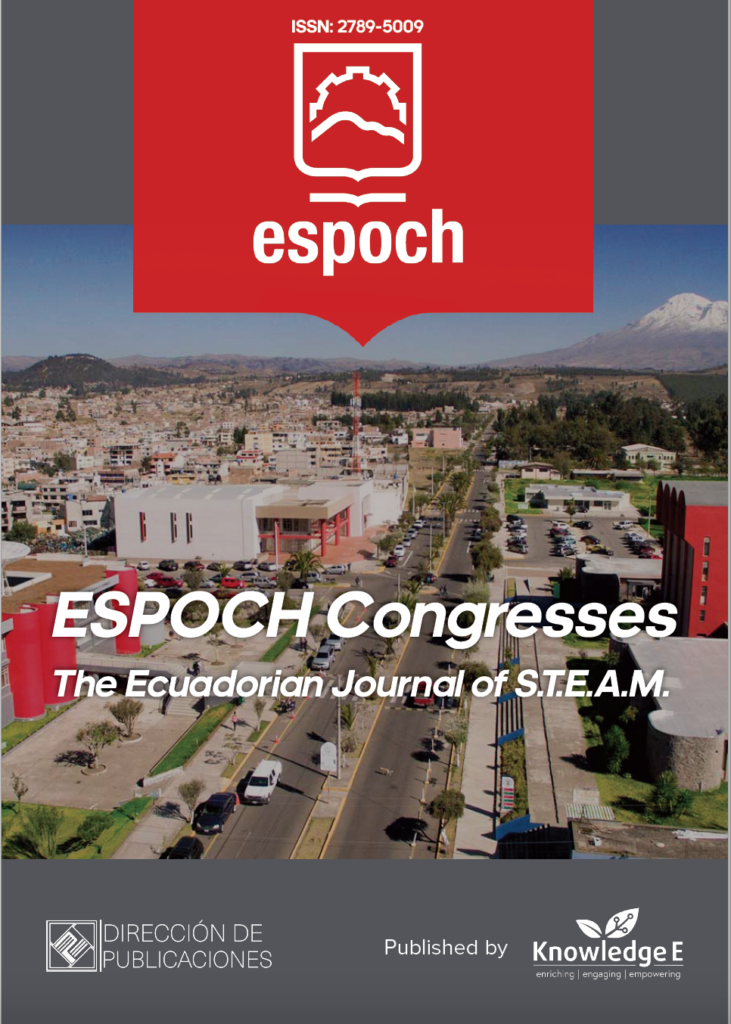
ESPOCH Congresses: The Ecuadorian Journal of S.T.E.A.M.
ISSN: 2789-5009
Leading Ecuadorian research in science, technology, engineering, arts, and mathematics.
Analysis of the Corrosion Resistance of Bronze to Aluminium (ASTM B 824) in a Corrosive Environment Controlled with an Artificial Seawater Solution
Published date: Aug 26 2021
Journal Title: ESPOCH Congresses: The Ecuadorian Journal of S.T.E.A.M.
Issue title: Volume 1, Issue 1
Pages: 175–194
Authors:
Abstract:
This paper presents the analysis of corrosion resistance of bronzes to aluminum in a controlled corrosive environment. Three alloys were studied CuAl4.5; CuAl7,1 and CuAl10,1 (ASTM B824), whose chemical composition was evaluated by spectrometry (OES). To determine its metal phases, chemical attacks were carried out with FeCl3, HCl in 95% Ethanol and FeCl3, HCl, CrO3 in distilled water. The microstructures obtained were characterized by metallography using two microscopes, an optical and a scanning electron (SEM) and the phases obtained were compared. Subsequently, electrochemical corrosion tests were performed on each alloy. The electrolyte used in the tests was artificial seawater (ASTM D1141) with a pH of 10 ± 0.3. Then, the corrosion products were characterized by EDS and SEM. Once the identification phase was over, the products were removed with a 50% HCl solution. Corrosive attack damage in each microstructural matrix was identified and corrosion rates for each alloy were evaluated. Finally, the corrosion rate data were correlated with the Al and Sn percentages of the alloy. The results show that the higher the increase in aluminum, the lower the corrosion rate, for a maximum limit of Al = 10.11%; Sn = 0.13%; CR = 5,170 mpy; In addition, it was shown that these alloys are effective for marine environments with high salinity. The correlation can be used to estimate the corrosion rate for different pH of the electrolytic medium of any type of ferrous or non-ferrous alloy whose variables are dependent on its chemical composition.
Keywords: corrosion, alloy, metallography, microstructure, spectrometry, electrochemistry.
Resumen
Este artículo presenta el análisis la resistencia a la corrosión de bronces al aluminio en un ambiente corrosivo controlado. Se estudiaron tres aleaciones CuAl4,5; CuAl7,1 y CuAl10,1 (ASTM B824), cuya composición química fue evaluada por espectrometría (OES). Para determinar sus fases metálicas se realizaron ataques químicos con FeCl3, HCl en Etanol al 95% y FeCl3, HCl, CrO3 en agua destilada. Las microestructuras obtenidas se caracterizaron mediante metalografía empleando dos microscopios, un óptico y un electrónico de barrido (SEM) y se compararon las fases obtenidas. Posteriormente, se realizaron ensayos de corrosión electroquímica a cada aleación. El electrolito utilizado en los ensayos fue agua de mar artificial (ASTM D1141) con un pH 10±0.3. Sucesivamente, se caracterizaron los productos de la corrosión mediante microscopia SEM. Una vez terminada la fase de identificación, se removieron los productos con una solución al 50% HCl. Los daños del ataque corrosivo en cada matriz microestructural fueron identificados y las tasas de corrosión para cada aleación fueron evaluadas. Finalmente, se correlacionaron los datos de tasas de corrosión con los porcentajes de Al y Sn de la aleación. Los resultados muestran que a mayor aumento de aluminio existe una menor tasa de corrosión, para un límite máximo de Al=10,11%; Sn=0.13%; CR=5,170 mpy; además, se demostró que estas aleaciones son eficaces para ambientes marinos con alta salinidad. La correlación puede ser utilizada para estimar la tasa de corrosión para diferentes pH del medio electrolítico de cualquier tipo de aleación ferrosa o no ferrosa cuyas variables sean dependientes de su composición química.
Palabras claves: corrosión, aleación, metalografía, microestructura, espectrometría, electroquímica.
References:
[1] Huttunen ES, Isotahdon E, Metsajoki J. Tribocorrosion behaviour of aluminium bronze in 3.5 wt.% NaCl solution. Corrosion Science. 2018;144:207-223.
[2] Fateh A, Aliofkhazraei MRAR. Review of corrosive environments for copper and its corrosion inhibitors. Arabian Journal of Chemistry. 2017;1-194.
[3] Tuthill AH. Materials performance focus on seawater corrosion. NACE. 1987; 1-12.
[4] Kadhum AAH, Mohamad AB, Jaffar HD et al. Corrosion of nickel-aluminum - bronze alloy in aerated 0.1 M sodium chloride solutions under hydrodynamic condition. International Journal of Electrochemical Science. 2013;8(4):11.
[5] Geler E, Azambuja DS. Corrosion inhibition of copper in chloride solutions by pyrazole. Corrosion Science. 2000;42(4):631-643.
[6] Genrengi H, Slepski P, Bereket G. Dynamic electrochemical impedance spectroscopy and polarization studies to evaluate the inhibition effect of benzotriazole on copper-manganese-aluminium alloy in artificial seawater. Materials and Corrosion. 2012;14(64):63.
[7] Hoar TP. Report of The commmittee on corrosion and protection. London: Dep. Of Trade and Industry, HMSO; 1971.
[8] Cargua-López MV. Optimización de la resistencia a la corrosión de un bronce al aluminio en un ambiente corrosivo controladoOptimización de la resistencia a la corrosión de un bronce al aluminio en un ambiente corrosivo controlado. Riobamba: Escuela Superior Politécnica de Chimborazo; 2019.
[9] Klement JF, M. R. E. Corrosion. 1960.
[10] Morral FR, Jimeo E, MP. Metalurgia general. Barcelona: Reverté, S.A; 1985.
[11] Wharton JA, Barik RG, Kear G. 2005. The corrosion of nickel-aluminium bronze in seawater. Corrosion Science. 2005;47:3336-3367.
[12] Montecinos S, Simison, SN. Influence of the microestructure on corrosion behaviour of a shape memory Cu-Al-Be alloy in marine evironment. n 2010;257:2737-2744.
[13] Stansbury EE, Buchanan RA. Fundaments of electrochemical corrosion. Tennessee: ASM International; 2000.
[14] Popo BN. Corrosion enginieering principles and solved problem. Oxford: Elsevier; 2015.
[15] Shalaby MN, Osman MM. Protection of copper surface against corrosion by cationic surfactant in seawater. Journal of Dispersion Science and Technology. 2009;677-683.
[16] Mansfeld F, Litte B. The Corrosion behavior of stainless steels and copper alloys exposed to natural seawater. Corrosion Science. 1991;36(0704- 0168):331-340.
[17] Park KS, Kim S. Corrosion and corrosion fatigue characteristics of cast NAB coated with NAB by HVOF Thermal Spray. Journal of The Electrochemical Society. 2011;10(158):C335-C340.
[18] Sullivan JC, Wong LF. Wear of aluminium bronze on steel under conditions of boundary lubrication. Tribology International. 1985;18:275- 281.
[19] Souto RM, González S, Salvarezza RC, Arvia AJ. Kinetics of copper passivation and pitting corrosion in Na2SO4 containing dilute NaOH aqueous solution. Electrochemical Act. 1986;39(17):2619-2628.
[20] Cornwell FJ, Wildsmith G, Gilbert, PT. Pitting corrosion in copper tubes in cold water service. British Corrosion Journal. 2013;8(5):202-209.
[21] Tromans D, Sun RG. Intergranular/transgranular fatigue of copper: Influence of environment on crack path and propagation rates. Materials Science and Engineering. 1996;219(1-2):56-65.
[22] Montgomery DC, Runger GC. Applied statidtics and probability for engineers. Limusa: Wiley; 2018.
[23] Almeida MB, Erthal RS, Padua EO, Silveira LV, Escaleira LA. Response surface methodology (RSM) as a tool for optimization in analytical chemistry. Talanta. 2008;76(5):965-977.