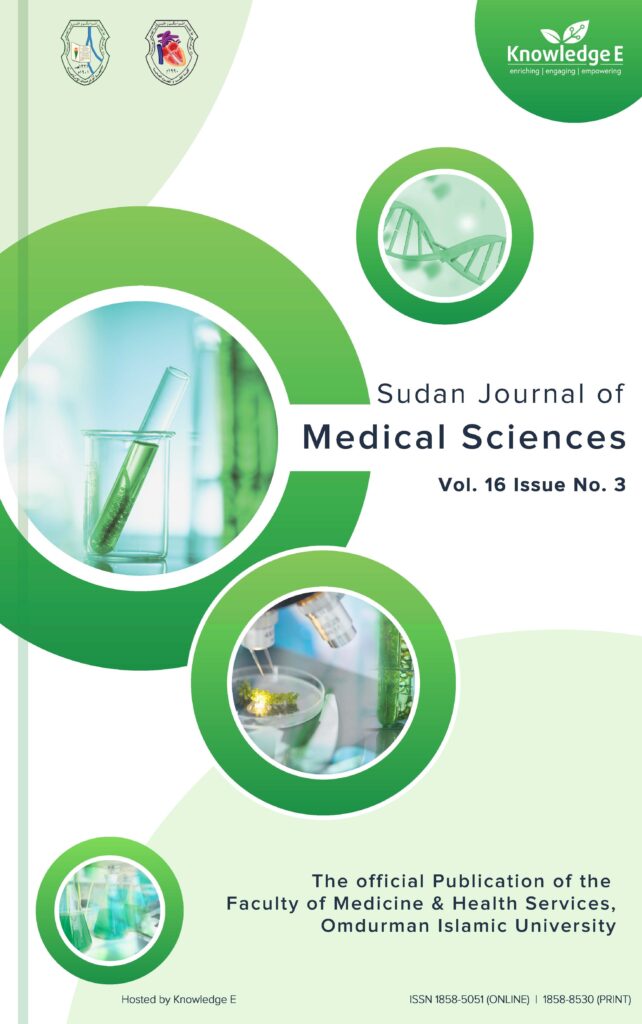
Sudan Journal of Medical Sciences
ISSN: 1858-5051
High-impact research on the latest developments in medicine and healthcare across MENA and Africa
Computational Screening of Repurposed Drugs Targeting Sars-Cov-2 Main Protease By Molecular Docking
Published date: Sep 30 2022
Journal Title: Sudan Journal of Medical Sciences
Issue title: Sudan JMS: Volume 17 (2022), Issue No. 3
Pages: 387 – 400
Authors:
Abstract:
Background: COVID-19 (Coronavirus disease 2019) is caused by the severe acute respiratory syndrome coronavirus type 2 (SARS-CoV-2), which poses significant global health and economic crisis that urges effective treatment.
Methods: A total of 11 molecules (baricitinib, danoprevir, dexamethasone, hydroxychloroquine, ivermectin, lopinavir, methylprednisolone, remdesivir, ritonavir and saridegib, ascorbic acid, and cepharanthine) were selected for molecular docking studies using AutoDock VINA to study their antiviral activities via targeting SARS-CoV’s main protease (Mpro), a cysteine protease that mediates the maturation cleavage of polyproteins during virus replication.
Results: Three drugs showed stronger binding affinity toward Mpro than N3 (active Mpro inhibitor as control): danoprevir (–7.7 kcal/mol), remdesivir (–8.1 kcal/mol), and saridegib (–7.8 kcal/mol). Two primary conventional hydrogen bonds were identified in the danoprevir-Mpro complex at GlyA:143 and GlnA:189, whereas the residue GluA:166 formed a carbon–hydrogen bond. Seven main conventional hydrogen bonds were identified in the remdesivir at AsnA:142, SerA:144, CysA:145, HisA:163, GluA:166, and GlnA:189, whereas two carbon–hydrogen bonds were formed by the residues HisA:41 and MetA:165. Cepharanthine showed a better binding affinity toward Mpro (–7.9 kcal/mol) than ascorbic acid (–5.4 kcal/mol). Four carbon–hydrogen bonds were formed in the cepharanthine-Mpro complex at HisA:164, ProA;168, GlnA;189, and ThrA:190.
Conclusion: The findings of this study propose that these drugs are potentially inhibiting the SAR-CoV-2 virus by targeting the Mpro protein.
Keywords: repurposed drug, COVID-19, Mpro, docking
References:
[1] Worldometer. (2021). Coronavirus updates. Worldometer.
[2] Guo, Y. R., Cao, Q. D., Hong, Z. S., Tan, Y. Y., Chen, S. D., Jin, H. J., Tan, K. S., Wang, D. Y., & Yan, Y. (2020). The origin, transmission and clinical therapies on coronavirus disease 2019 (COVID-19) outbreak - An update on the status. Military Medical Research, 7, 11. https://doi.org/10.1186/s40779-020-00240-0
[3] Mathieu, E., Ritchie, H., Ortiz-Ospina, E., Roser, M., Hasell, J., Appel, C., Giattino, C., & Rodés-Guirao, L. (2021). A global database of COVID-19 vaccinations. Nature Human Behaviour, 5, 947–953. https://doi.org/10.1038/s41562-021-01122-8
[4] Moghadas, S. M., Vilches, T. N., Zhang, K., Wells, C. R., Shoukat, A., Singer, B. H., Meyers, L. A., Neuzil, K. M., Langley, J. M., Fitzpatrick, M. C., & Galvani, A. P. (2021). The impact of vaccination on coronavirus disease 2019 (COVID- 19) outbreaks in the United States. Clinical Infectious Diseases, 73, 2257–2264. https://doi.org/10.1093/cid/ciab079
[5] Mallapaty, S., Callaway, E., Kozlov, M., Ledford, H., Pickrell, J., & Van Noorden, R. (2021). How COVID vaccines shaped 2021 in eight powerful charts. Nature, 600, 580–583. https://doi.org/10.1038/d41586-021-03686-x
[6] Pushpakom, S., Iorio, F., Eyers, P. A., Escott, K. J., Hopper, S., Wells, A., Doig, A., Guilliams, T., Latimer, J., McNamee, C., Norris, A., Sanseau, P., Cavalla, D., & Pirmohamed, M. (2019). Drug repurposing: Progress, challenges and recommendations. Nature Reviews. Drug Discovery, 18, 41–58. https://doi.org/10.1038/nrd.2018.168
[7] Parvathaneni, V., & Gupta, V. (2020). Utilizing drug repurposing against COVID-19 - Efficacy, limitations, and challenges. Life Sciences, 259, 118275. https://doi.org/10.1016/j.lfs.2020.118275
[8] Zhang, S. (2011). Computer-aided drug discovery and development. Drug Design and Discovery, 716, 23–38. https://doi.org/10.1007/978-1-61779-012-6_2
[9] Wang, M.-Y., Zhao, R., Gao, L.-J., Gao, X.-F., Wang, D.-P., & Cao, J.- M. (2020). SARS-CoV-2: Structure, biology, and structure-based therapeutics development. Frontiers in Cellular and Infection Microbiology, 10, 587269. https://doi.org/10.3389/fcimb.2020.587269
[10] Prajapat, M., Sarma, P., Shekhar, N., Avti, P., Sinha, S., Kaur, H., Kumar, S., Bhattacharyya, A., Kumar, H., Bansal, S., & Medhi, B. (2020). Drug targets for corona virus: A systematic review. Indian Journal of Pharmacology, 52, 56–65. https://doi.org/10.4103/ijp.IJP_115_20
[11] Khan, S. A., Zia, K., Ashraf, S., Uddin, R., & Ul-Haq, Z. (2021). Identification of chymotrypsin-like protease inhibitors of SARS-CoV-2 via integrated computational approach. Journal of Biomolecular Structure & Dynamics, 39, 2607–2616. https://doi.org/10.1080/07391102.2020.1751298
[12] Jin, Z., Du, X., Xu, Y., Deng, Y., Liu, M., Zhao, Y., Zhang, B., Li, X., Zhang, L., Peng, C., Duan, Y., Yu, J., Wang, L., Yang, K., Liu, F., Jiang, R., Yang, X., You, T., Liu, X., … Yang, H. (2020). Structure of Mpro from SARS-CoV-2 and discovery of its inhibitors. Nature, 582, 289–293. https://doi.org/10.1038/s41586-020-2223-y
[13] Lovell, S. C., Davis, I. W., Arendall, W. B., III, de Bakker, P. I. W., Word, J. M., Prisant, M. G., Richardson, J. S., & Richardson, D. C. (2003). Structure validation by Calpha geometry: Phi,ψ and Cbeta deviation. Proteins, 50, 437–450. https://doi.org/10.1002/prot.10286
[14] Trott, O., Olson, A. J. J. (2010). AutoDock Vina: Improving the speed and accuracy of docking with a new scoring function, efficient optimization, and multithreading. Journal of Computational Chemistry, 31(2), 455-461.
[15] Dassault Systemes. (2017). BIOVIA. BIOVIA Discovery Studio Visualizer.
[16] Huynh, T., Wang, H., Luan, B. (2020). In silico exploration of the molecular mechanism of clinically oriented drugs for possibly inhibiting SARS-CoV-2’s main protease. Journal of Physical Chemistry Letters, 11(11), 4413–4420.
[17] Kanhed, A. M., Patel, D. V., Teli, D. M., Patel, N. R., Chhabria, M. T., Yadav, M.R.J.M.d. (2021). Identification of potential Mpro inhibitors for the treatment of COVID-19 by using systematic virtual screening approach. Molecular Diversity, 25(1), 383–401.
[18] Jeffrey, G. A., & Saenger, W. (2012). Hydrogen bonding in biological structures. Springer Science & Business Media.
[19] Meyer, E. E., Rosenberg, K. J., Israelachvili, J. (2006). Recent progress in understanding hydrophobic interactions. PNAS, 103(43), 15739–15746.
[20] Harisna, A. H., Nurdiansyah, R., Syaifie, P. H., Nugroho, D. W., Saputro, K. E., Firdayani., Prakoso, C. D., Rochman, N. T., Maulana, N. N., Noviyanto, A., & Mardliyati, E. (2021). In silico investigation of potential inhibitors to main protease and spike protein of SARS-CoV-2 in propolis. Biochemistry and Biophysics Reports, 26, 100969. https://doi.org/10.1016/j.bbrep.2021.100969
[21] Kumar, K., Woo, S. M., Siu, T., Cortopassi, W. A., Duarte, F., & Paton, R. S. (2018). Cation-π interactions in protein-ligand binding: Theory and data-mining reveal different roles for lysine and arginine. Chemical Science (Cambridge), 9, 2655– 2665. https://doi.org/10.1039/C7SC04905F
[22] Ferreira de Freitas, R., & Schapira, M. (2017). A systematic analysis of atomic protein-ligand interactions in the PDB. MedChemComm, 8, 1970–1981. https://doi.org/10.1039/C7MD00381A
[23] Miao, M., Jing, X., De Clercq, E., & Li, G. (2020). Danoprevir for the treatment of hepatitis C virus infection: Design, development, and place in therapy. Drug Design, Development and Therapy, 14, 2759–2774. https://doi.org/10.2147/DDDT.S254754
[24] Hosseini, F. S., & Amanlou, M. (2020). Simeprevir, potential candidate to repurpose for coronavirus infection: Virtual screening and molecular docking study. Preprint.org. https://www.preprints.org/manuscript/202002.0438/v1
[25] da Costa, L. J., Pereira, M. M., de Souza Ramos, L., de Mello, T. P., Silva, L. N., Branquinha, M. H., & Dos Santos, A. L. S. (2021). Protease Inhibitors as Promising Weapons against COVID-19: Focus on repurposing of drugs used to treat HIV and HCV infections. Current Topics in Medicinal Chemistry, 21, 1429–1438. https://doi.org/10.2174/1568026621666210701093407
[26] Chen, H., Zhang, Z., Wang, L., Huang, Z., Gong, F., Li, X., Chen, Y., & Wu, J. J. (2020). First clinical study using HCV protease inhibitor danoprevir to treat COVID- 19 patients. Medicine, 99, e23357. https://doi.org/10.1097/MD.0000000000023357
[27] Vangeel, L., Chiu, W., De Jonghe, S., Maes, P., Slechten, B., Raymenants, J., André, E., Leyssen, P., Neyts, J., & Jochmans, D. (2022). Remdesivir, Molnupiravir and Nirmatrelvir remain active against SARS-CoV-2 Omicron and other variants of concern. Antiviral Research, 198, 105252. https://doi.org/10.1016/j.antiviral.2022.105252
[28] Kokic, G., Hillen, H. S., Tegunov, D., Dienemann, C., Seitz, F., Schmitzova, J., Farnung, L., Siewert, A., Höbartner, C., & Cramer, P. (2021). Mechanism of SARS-CoV-2 polymerase stalling by remdesivir. Nature Communications, 12, 279. https://doi.org/10.1038/s41467-020-20542-0
[29] Naik, V. R., Munikumar, M., Ramakrishna, U., Srujana, M., Goudar, G., Naresh, P., Kumar, B. N., & Hemalatha, R. (2021). Remdesivir (GS-5734) as a therapeutic option of 2019-nCOV main protease - In silico approach. Journal of Biomolecular Structure & Dynamics, 39, 4701–4714. https://doi.org/10.1080/07391102.2020.1781694
[30] Nguyen, H. L., Thai, N. Q., Truong, D. T., & Li, M. S. (2020). Remdesivir strongly binds to both RNA-dependent RNA polymerase and main protease of SARS-CoV- 2: Evidence from molecular simulations. The Journal of Physical Chemistry B, 124, 11337–11348. https://doi.org/10.1021/acs.jpcb.0c07312
[31] Beigel, J. H., Tomashek, K. M., Dodd, L. E., Mehta, A. K., Zingman, B. S., Kalil, A. C., Hohmann, E., Chu, H. Y., Luetkemeyer, A., Kline, S., Lopez de Castilla, D., Finberg, R. W., Dierberg, K., Tapson, V., Hsieh, L., Patterson, T. F., Paredes, R., Sweeney, D. A., Short, W. R., … Lane, H. C., & the ACTT-1 Study Group Members. (2020). Remdesivir for the treatment of Covid-19 - Final report. The New England Journal of Medicine, 383, 1813–1826. https://doi.org/10.1056/NEJMoa2007764
[32] Smelkinson, M. G. (2017). The Hedgehog signaling pathway emerges as a pathogenic target. Journal of Developmental Biology, 5, 14. https://doi.org/10.3390/jdb5040014
[33] Baratella, E., Bussani, R., Zanconati, F., Marrocchio, C., Fabiola, G., Braga, L., Maiocchi, S., Berlot, G., Volpe, M. C., Moro, E., Confalonieri, P., Cova, M. A., Confalonieri, M., Salton, F., & Ruaro, B. (2021). Radiological-pathological signatures of patients with COVID-19-related pneumomediastinum: Is there a role for the Sonic hedgehog and Wnt5a pathways? ERJ Open Research, 7, 7. https://doi.org/10.1183/23120541.00346- 2021
[34] Lee, M. J., Hatton, B. A., Villavicencio, E. H., Khanna, P. C., Friedman, S. D., Ditzler, S., Pullar, B., Robison, K., White, K. F., Tunkey, C., LeBlanc, M., Randolph- Habecker, J., Knoblaugh, S. E., Hansen, S., Richards, A., Wainwright, B. J., McGovern, K., & Olson, J. M. (2012). Hedgehog pathway inhibitor saridegib (IPI- 926) increases lifespan in a mouse medulloblastoma model. Proceedings of the National Academy of Sciences of the United States of America, 109, 7859–7864. https://doi.org/10.1073/pnas.1114718109
[35] Watt, M. M., Collins, M. S., & Johnson, D. W. (2013). Ion-π interactions in ligand design for anions and main group cations. Accounts of Chemical Research, 46, 955–966. https://doi.org/10.1021/ar300100g
[36] Schottel, B. L., Chifotides, H. T., & Dunbar, K. R. (2008). Anion-π interactions. Chemical Society Reviews, 37, 68–83. https://doi.org/10.1039/B614208G
[37] Rogosnitzky, M., Okediji, P., & Koman, I. (2020). Cepharanthine: A review of the antiviral potential of a Japanese-approved alopecia drug in COVID-19. Pharmacological Reports, 72, 1509–1516.
[38] Mani, J. S., Johnson, J. B., Steel, J. C., Broszczak, D. A., Neilsen, P. M., Walsh, K. B., & Naiker, M. (2020). Natural product-derived phytochemicals as potential agents against coronaviruses: A review. Virus Research, 284, 197989. https://doi.org/10.1016/j.virusres.2020.197989
[39] Ohashi, H., Watashi, K., Saso, W., Shionoya, K., Iwanami, S., Hirokawa, T., Shirai, T., Kanaya, S., Ito, Y., Kim, K. S., Nomura, T., Suzuki, T., Nishioka, K., Ando, S., Ejima, K., Koizumi, Y., Tanaka, T., Aoki, S., Kuramochi, K., … Wakita, T. (2021). Potential anti- COVID-19 agents, cepharanthine and nelfinavir, and their usage for combination treatment. iScience, 24, 102367. https://doi.org/10.1016/j.isci.2021.102367
[40] Ruan, Z., Liu, C., Guo, Y., He, Z., Huang, X., Jia, X., & Yang, T. (2021). SARSCoV- 2 and SARS-CoV: Virtual screening of potential inhibitors targeting RNA-dependent RNA polymerase activity (NSP12). Journal of Medical Virology, 93, 389– 400. https://doi.org/10.1002/jmv.26222
[41] Bauer, S. R., Kapoor, A., Rath, M., & Thomas, S. A. (2020). What is the role of supplementation with ascorbic acid, zinc, vitamin D, or N-acetylcysteine for prevention or treatment of COVID-19? Cleveland Clinic Journal of Medicine. https://doi.org/10.3949/ccjm.87a.ccc046