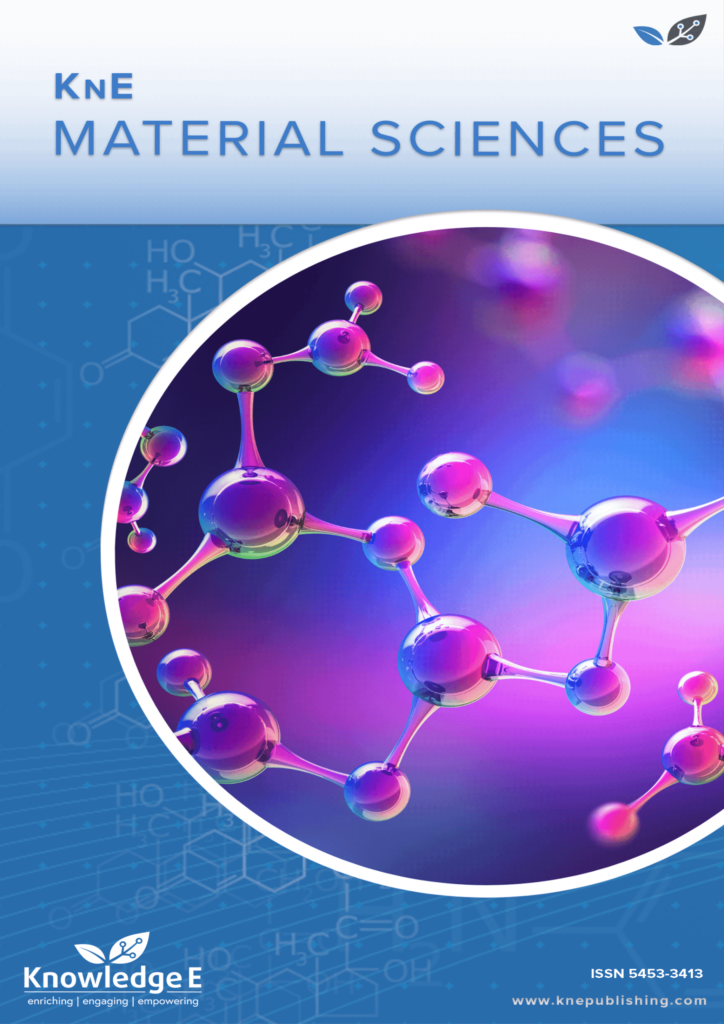
KnE Materials Science
ISSN: 2519-1438
The latest conference proceedings on physical materials, energy materials, electrical materials.
Heating Kinetics Simulation During Spark-Plasma Sintering of Non-Conductive Materials
Published date: May 06 2018
Journal Title: KnE Materials Science
Issue title: 15th International School-Conference "New Materials – Materials of Innovative Energy" (MIE)
Pages: 575–585
Authors:
Abstract:
The simulation of aluminum oxide spark-plasma sintering has been carried out during this research, namely the temperature field distribution in the sample volume and mold at different heating phases. The research work was based on experimental data on the measurement of temperature on the matrix surface, in its hole and various internal parts, including the punches, in the absence of thermal insulating felt. It was experimentally discovered that the key source of heat release up to temperatures 1300-1400 ∘C is the contact resistance at the matrix punch boundaries. Then the heat dissipation zone moves towards the punches. The collected data helped to figure out thermal and electrical parameters for the materials used in the die mold. They provided a good coincidence of the observed and calculated figures of heat distribution at different temperatures. The parameters helped to calculate the temperature fields in the die molds with heat-insulating felts.
Keywords: spark-plasma sintering, finite element modeling, temperature distribution, electric and thermal contact.
References:
[1] R. Orrù, R. Licheri, A.M. Locci, A. Cincotti, G. Cao, Consolidation/synthesis of materials by electric current activated/assisted sintering, Mater. Sci. Eng. R 63 (4–6) (2009) 127–287.
[2] O. Guillon, J.G. Julian, B. Dargatz, T. Kessel, G. Schierning, J. Räthel, M. Herrmann, Field-assisted sintering technology/spark plasma sintering: mechanisms, materials, and technology developments, Adv. Eng. Mater. 16 (7) (2014) 830–849.
[3] M. Suárez, A. Fernández, J.L. Menéndez, R. Torrecillas, H.U. Kessel, J. Hennicke, R. Kirchner, T. Kessel, Chapter 13: Challenges and opportunities for spark plasma sintering: a key technology for a new generation of materials, in: B. Ertuğ (Ed.), Sintering Applications, InTech, ISBN: 978-953-51-0974-7 2013, pp. 319–342
[4] Z.A. Munir, U. Anselmi-Tamburini, M. Ohyanagi, The effect of electric field and pressure on the synthesis and consolidation of materials: a review of the spark plasma sintering method, J. Mater. Sci. 41 (2006) 763–777.
[5] A. Pavia, L. Durand, F. Ajustron, V. Bley, G. Chevallier, A. Peigney, C. Estournès, Electro-thermal measurements and finite element method simulations of a spark plasma sintering device, J. Mater. Process. Technol. 213 (8) (2013) 1327–1336.
[6] L. Junting, S. Yan, Z. Chunxiang, Z. Zhiyong, Thermal-electrical coupled analysis and experimental investigation on spark plasma sintering of SiC ceramics, J. Wuhan Univ. Technol. - Mater. Sci. Ed. 27 (6) (2012) 1120–1124.
[7] C. Manière, A. Pavia, L. Durand, G. Chevallier, K. Afanga, C. Estournès Finite-element modeling of the electro-thermal contacts in the spark plasma sintering process, J. Eur. Ceram. Soc.,36 (2016) 741–748.
[8] A. Zavaliangos, J. Zhang, M. Krammer, J.R. Groza, Temperature evolution during field activated sintering, Mater. Sci. Eng. A 379 (1–2) (2004) 218–228.
[9] K. Vanmeensel, A. Laptev, J. Hennicke, J. Vleugels, O. Van der Biest, Modelling of the temperature distribution during field assisted sintering, Acta Mater. 53 (16) (2005) 4379–4388.
[10] X. Wang, S.R. Casolco, G. Xu, J.E. Garay, Finite element modeling of electric currentactivated sintering: the effect of coupled electrical potential, temperature and stress, Acta Mater. 55 (10) (2007) 3611–3622.
[11] B. McWilliams, A. Zavaliangos, Multi-phenomena simulation of electric field assisted sintering, J. Mater. Sci. 43 (14) (2008) 5031–5035.
[12] S. Muñoz, U. Anselmi-Tamburini, Temperature and stress fields evolution during spark plasma sintering processes, J. Mater. Sci. 45 (23) (2010) 6528–6539.
[13] P. Mondalek, L. Silva, M. Bellet, A numerical model for powder densification by SPS technique, Adv. Eng. Mater. 13 (7) (2011) 587–593.
[14] G. Antou, G. Mathieu, G. Trolliard, A. Maître, Spark plasma sintering of zirconium carbide and oxycarbide: finite element modeling of current density, temperature, and stress distributions, J. Mater. Res. 24 (02) (2009) 404–412.
[15] G. Maizza, S. Grasso, Y. Sakka, Moving finite-element mesh model for aiding spark plasma sintering in current control mode of pure ultrafine WC powder, J. Mater. Sci. 44 (5) (2009) 1219–1236.
[16] S. Muñoz, U. Anselmi-Tamburini, Parametric investigation of temperature distribution in field activated sintering apparatus, Int. J. Adv. Manuf. Technol. 65 (1) (2013) 127–140.
[17] Y. Achenani, M. Saâdaoui, A. Cheddadi, G. Bonnefont, G. Fantozzi, Finite element modeling of spark plasma sintering: Application to the reduction of temperature inhomogeneities, case of alumina, Materials and Design 116 (2017) 504–514.
[18] C. Maniere, A. Pavia, L. Durand, G. Chevallier, V. Bley, K. Afanga, A. Peigney, C. Estournes, Pulse analysis and electric contact measurements in spark plasma sintering, Electr. Power Syst. Res. 127 (2015) 307–313.
[19] E.A. Olevsky, C. Garcia-Cardona, W.L. Bradbury, C.D. Haines, D.G. Martin, D. Kapoor, Fundamental aspects of spark plasma sintering II: finite element analysis of scalability, J. Am. Ceram. Soc. 95 (2012) 2414–2422.
[20] A. Locci, A. Cincotti, S. Todde, R. Orrù and G, Cao, A methodology to investigate the intrinsic effect of the pulsed electric current during the spark plasma sintering of electrically conductive powders, Sci. Technol. Adv. Mater.11 (4) (2010) 045005.
[21] C. Maniere, G. Lee, E. Olevsky, Proportional integral derivative, modeling and ways of stabilization for the spark plasma sintering process, Results in Physics, 7 (2017) 1494 – 1497.