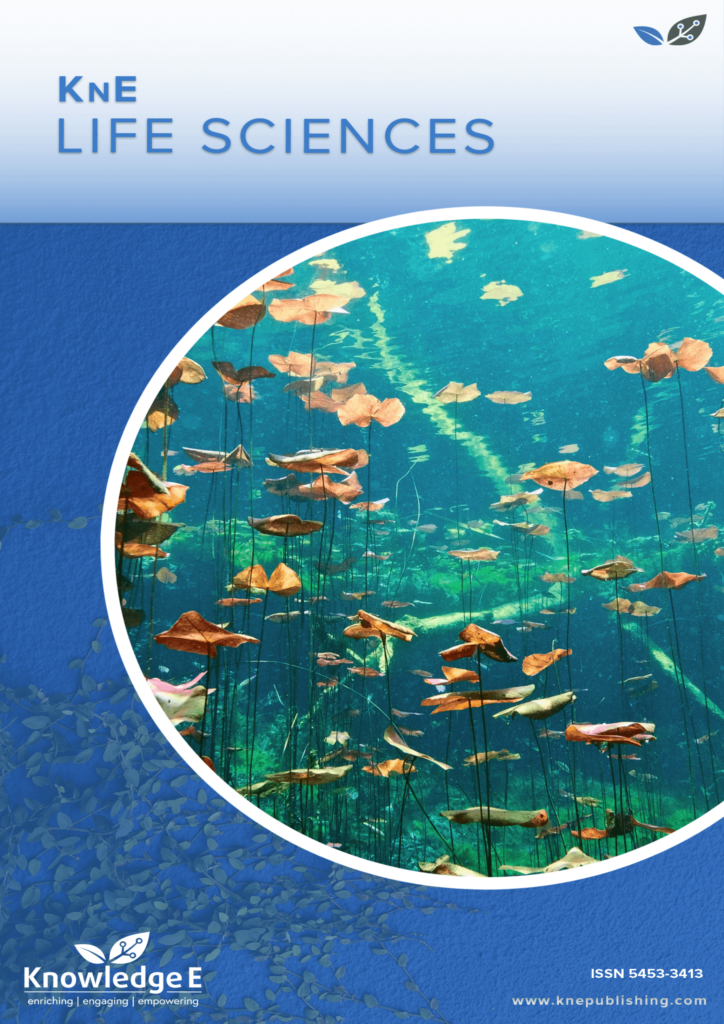
KnE Life Sciences
ISSN: 2413-0877
The latest conference proceedings on life sciences, medicine and pharmacology.
Investigating the Characteristics and Evolution of Apical Membrane Antigen 1 (AMA1) of Plasmodium sp. using Phylogenetic Approach in Searching for Drug Candidate
Published date: Feb 11 2020
Journal Title: KnE Life Sciences
Issue title: The 2019 International Conference on Biotechnology and Life Sciences (IC-BIOLIS)
Pages:
Authors:
Abstract:
Malaria is an endemic disease caused by Plasmodium parasite with female Anopheles mosquitoes as a vector. With the increase of drug resistance Plasmodium emerging around the world, a new method should be devised against the spread of Plasmodium sp lineage. Protein evolution of Plasmodium sp. can be an invaluable aid as an input for rational drug design and immune-informatics methods based on the novel virulent gene that exists in these protozoa. Previously, we did data mining in the PlasmoDB database and found several proteins shared by Plasmodium, one of them was the Apical Membrane Antigen 1 (AMA1). This protein was further analyzed for its characteristics and evolutionary properties. AMA1 protein sequences from human-infecting Plasmodium (P. falciparum, vivax, knowlesi, ovale, and malariae) and non-infectious (P. berghei, coatneyi, and cynomolgi) were retrieved from PlasmoDB. Maximum likelihood phylogenetic tree with molecular clock analysis was reconstructed from AMA1 sequences using MEGAX. Protein domain analysis was done using the INTERPRO server. Several domains that can induce protective immunity and vaccine target were found in AMA1 of those plasmodium, such as coiled-coil, disordered region, and signal peptide domain. Furthermore, molecular clock analysis showed a similar evolutionary rate between AMA1 protein in human-infecting and non-infectious Plasmodium. Interestingly, the phylogenetic tree showed a mixed cluster of human- infecting with non-infectious, indicating a unique evolutionary relationship between Plasmodium lineage. Thus, this information could be beneficial in developing the drug and vaccine for Plasmodium-related infection.
Keywords: AMA1, drug target, protein domain, protein evolution, Plasmodium.
References:
[1] A. Y. Vittor et al., “Linking Deforestation to Malaria in the Amazon: Characterization of the Breeding Habitat of the Principal Malaria Vector, Anopheles darlingi,” Am J Trop Med Hyg, vol. 10, no. 1, pp. 54–56, 2013.
[2] World Health Organization, World Malaria Report 2018. 2018.
[3] H. Noedl, Y. Se, K. Schaecher, B. L. Smith, D. Socheat, and M. M. Fukuda, “Evidence of artemisinin- resistant malaria in Western Cambodia,” N. Engl. J. Med., vol. 359, no. 24, pp. 2619–2620, 2008.
[4] R. Amato et al., “Origins of the current outbreak of multidrug-resistant malaria in southeast Asia: a retrospective genetic study,” Lancet Infect. Dis., vol. 18, no. 3, pp. 337–345, 2018.
[5] World Health Organization, “Artemisinin resistance and artemisinin-based combination therapy efficacy,” 2018.
[6] G. H. Mitchell, A. W. Thomas, G. Margos, A. R. Dluzewski, and L. H. Bannister, “Apical Membrane Antigen 1, a Major Malaria Vaccine Candidate, Mediates the Close Attachment of Invasive Merozoites to Host Red Blood Cells,” Infect. Immun., vol. 72, no. 1, pp. 154–158, 2004.
[7] T. Triglia et al., “Apical membrane antigen 1 plays a central role in erythrocyte invasion by Plasmodium species,” Mol. Microbiol., vol. 38, no. 4, pp. 706–718, 2000.
[8] D. R. Drew et al., “Defining the Antigenic Diversity of Plasmodium falciparum Apical Membrane Antigen 1 and the Requirements for a Multi-Allele Vaccine against Malaria,” PLoS One, vol. 7, no. 12, 2012.
[9] F. Jahangiri, N. Jalallou, and M. Ebrahimi, “Analysis of Apical Membrane Antigen (AMA)-1 characteristics using bioinformatics tools in order to vaccine design against Plasmodium vivax,” Infect. Genet. Evol., vol. 71, no. March, pp. 224–231, 2019.
[10] M. Akter et al., “Identification of the Binding Site of Apical Membrane Antigen 1 (AMA1) Inhibitors Using a Paramagnetic Probe,” ChemMedChem, vol. 1, 2019.
[11] A. Ebrahimzadeh, A. Gharaei, and K. Saryazdi, “Allelic Diversity of Polymorphic AMA-1 (Apical Membrane Antigen 1) Vaccine Candidate Antigen of Plasmodium falciparum in Two Population of Imported and Indigenous Cases in South-East of Iran using Nested-PCR and RFLP,” J. Trop. Dis., vol. 02, no. 05, pp. 5–8, 2014.
[12] A. Gilabert et al., “Plasmodium vivax-like genome sequences shed new insights into Plasmodium vivax biology and evolution,” PLoS Biol., vol. 16, no. 8, pp. 1–25, 2018.
[13] G. G. Rutledge et al., “Plasmodium malariae and P. ovale genomes provide insights into malaria parasite evolution,” Nature, vol. 542, no. 7639, pp. 101–104, 2017.
[14] S. Tachibana et al., “Plasmodium cynomolgi genome sequences provide insight into Plasmodium vivax and the monkey malaria clade,” vol. 44, no. 9, pp. 1051–1055, 2013.
[15] B. G. Hall, “Building phylogenetic trees from molecular data with MEGA,” Mol. Biol. Evol., vol. 30, no. 5, pp. 1229–1235, 2013.
[16] S. Kumar, G. Stecher, M. Li, C. Knyaz, and K. Tamura, “MEGA X: Molecular evolutionary genetics analysis across computing platforms,” Mol. Biol. Evol., vol. 35, no. 6, pp. 1547–1549, 2018.
[17] R. C. Edgar, “MUSCLE: Multiple sequence alignment with high accuracy and high throughput,” Nucleic Acids Res., vol. 32, no. 5, pp. 1792–1797, 2004.
[18] B. Mello, “Estimating timetrees with MEGA and the timetree resource,” Mol. Biol. Evol., vol. 35, no. 9, pp. 2334–2342, 2018.
[19] S. B. Hedges, J. Marin, M. Suleski, M. Paymer, and S. Kumar, “Tree of life reveals clock-like speciation and diversification,” Mol. Biol. Evol., vol. 32, no. 4, pp. 835–845, 2015.
[20] A. G. Craig et al., “The role of animal models for research on severe malaria,” PLoS Pathog., vol. 8, no. 2, 2012.
[21] R. Basir et al., “Plasmodium berghei ANKA infection in ICR mice as a model of cerebral malaria,” Iran. J. Parasitol., vol. 7, no. 4, pp. 62–74, 2012.
[22] A. L. Goodman et al., “The utility of Plasmodium berghei as a rodent model for anti-merozoite malaria vaccine assessment,” Sci. Rep., vol. 3, 2013.
[23] C. H. M. Kocken et al., “Precise timing of expression of a Plasmodium falciparum-derived transgene in Plasmodium berghei is a critical determinant of subsequent subcellular localization,” J. Biol. Chem., vol. 273, no. 24, pp. 15119–15124, 1998.
[24] A. S. Othman et al., “Expression of full-length Plasmodium falciparum P48/45 in P. berghei blood stages: A method to express and evaluate vaccine antigens,” Mol. Biochem. Parasitol., vol. 224, no. July, pp. 44–49, 2018.
[25] A. Moreno et al., “Plasmodium coatneyi in rhesus macaques replicates the multisystemic dysfunction of severe malaria in humans,” Infect. Immun., vol. 81, no. 6, pp. 1889–1904, 2013.
[26] D. R. Drew, P. R. Sanders, G. Weiss, P. R. Gilson, B. S. Crabb, and J. G. Beeson, “Functional Conservation of the AMA1 Host-Cell Invasion Ligand between P. falciparum and P. vivax: A Novel Platform to Accelerate Vaccine and Drug Development,” J. Infect. Dis., vol. 217, no. 3, pp. 498–503, 2018.
[27] R. Kovjazin and L. Carmon, “The use of signal peptide domains as vaccine candidates,” Hum. Vaccines Immunother., vol. 10, no. 9, pp. 2733–2740, 2014.
[28] V. Villard et al., “Rapid identification of malaria vaccine candidates based on α-helical coiled coil protein motif,” PLoS One, vol. 2, no. 7, 2007.
[29] C. P. Karch et al., “The use of a P. falciparum specific coiled-coil domain to construct a self-assembling protein nanoparticle vaccine to prevent malaria,” J. Nanobiotechnology, vol. 15, no. 1, pp. 1–10, 2017.
[30] M. M. Babu, “The contribution of intrinsically disordered regions to protein function, cellular complexity, and human disease,” Biochem. Soc. Trans., vol. 44, no. 5, pp. 1185–1200, 2016.
[31] M. Madan Babu, R. Van Der Lee, N. Sanchez De Groot, J. Rg Gsponer, J. Gough, and K. Dunker, “Intrinsically disordered proteins: regulation and disease This review comes from a themed issue on Sequences and topology Edited,” Curr. Opin. Struct. Biol., vol. 21, pp. 1–9, 2011.
[32] J. H. Na, W. K. Lee, and Y. G. Yu, “How do we study the dynamic structure of unstructured proteins: A case study on nopp140 as an example of a large, intrinsically disordered protein,” Int. J. Mol. Sci., vol. 19, no. 2, 2018.
[33] E. R. Tamarozzi and S. Giuliatti, “Understanding the role of intrinsic disorder of viral proteins in the oncogenicity of different types of HPV,” Int. J. Mol. Sci., vol. 19, no. 1, 2018.