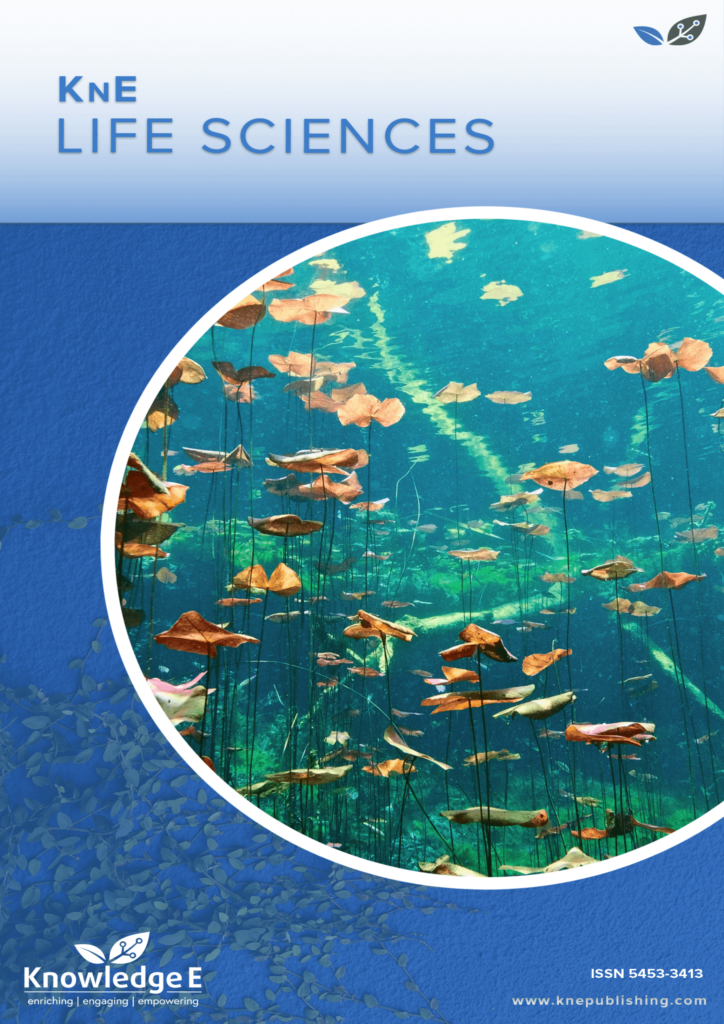
KnE Life Sciences
ISSN: 2413-0877
The latest conference proceedings on life sciences, medicine and pharmacology.
Bioinformatics Analyses of Endo-1,4-beta-xylanase from Bacillus sp. SS3.4
Published date: Feb 11 2020
Journal Title: KnE Life Sciences
Issue title: The 2019 International Conference on Biotechnology and Life Sciences (IC-BIOLIS)
Pages:
Authors:
Abstract:
Various types of microorganisms particularly bacteria and fungi are capable of producing xylanolytic enzymes that degrade xylan into simple sugars to be used as their carbon sources. Xylanolytic enzymes, particularly endo-1,4-β-xylanase, has been extensively used in various industrial sectors, including biofuel, livestock, paper, and food. Previously, Bacillus sp. SS3.4 was observed to have xylanolytic activities on wheat bran, suggesting its capability of producing xylanolytic enzymes, endo-1,4-β-xylanase included. With great numbers of data available in nucleotide and protein sequence databases, bioinformatics analyses of protein structure for function prediction have become attractive to supplement wet laboratory experiments to reduce time and money consumption. Therefore, in this study, bioinformatics analyses were performed on Bacillus sp. endo-1,4-β-xylanase sequence in order to further evaluate the enzyme’s function by exploring its protein structure in comparison to other known bacterial endo-1,4-β-xylanase. In this study, Bacillus sp. endo-1,4-β-xylanase sequence was identified from its whole genome sequence. The sequence was then analyzed by using BLAST to perform a homology search for identifying the protein, MUSCLE to perform multiple sequence alignment for comparing with other enzyme sequences, PHYLOGENY-FR to display its relatedness among Bacillus, Aspergillus niger and Trichoderma reesei, and SWISS-MODEL to generate its three-dimensional structure. Results from BLAST confirmed that the identified sequence was endo-1,4-β-xylanase, with greater relatedness to Bacillus velezensis and Bacillus amyloliquefaciens endo- 1,4-β-xylanase. While results from MUSCLE and SWISS-MODEL suggested that the endo-1,4-β-xylanase belongs to the Glycosyl Hydrolase 11 (GH11) family bearing the distinct shape of a jelly roll with well-conserved binding and catalytic residues. These results strongly suggest that Bacillus sp. endo-1,4-β-xylanase is potentially capable of degrading xylan with highly similar mechanism as with other endo-1,4-β-xylanase.
Keywords: Bacillus, bioinformatics analysis, endo-1,4-
References:
[1] T. Collins, C. Gerday, and G. Feller, ”Xylanases, xylanase families and extremophilic xylanases,” FEMS Microbiol. Rev., vol. 29, no. 1, pp. 3-23, 2005.
[2] P.J. Smith, H.T Wang, W.S. York, M.J. Peña, and B.R. Urbanowicz, “Designer biomass for next-generation biorefineries: Leveraging recent insights into xylan structure and biosynthesis,” Biotechnol. Biofuels, vol. 10, pp. 286, 2017.
[3] A.C.E.P. Benedetti, E.D. da Costa, C.C. Aragon, A.F. dos Santos, A.J. Goulart, D. Attili-Angelis, and R. Monti, ”Low-cost carbon sources for the production of a thermostable xylanase by Aspergillus niger,” Revista de Ciências Farmacêuticas Básica e Aplicada, vol. 34, no. 1, pp. 25.31, 2013.
[4] G. Paës, J.G. Berrin, and J. Beaugrand, ”GH11 xylanases: Structure/function/properties relationships and applications,”. Biotechnol. Adv., vol. 30, no. 3, pp. 564-592, 2012.
[5] A. Burlacu, C.P. Cornea, and F. Israel-Roming, ”Microbial xylanase: a review,” Scientific Bulletin Series
F. Biotechnologies, vol. 20, pp. 335-342, 2016.
[6] A.D. Harris, and C. Ramalingam, ”Xylanases and its application in food industry: A review,” J. Exp. Sci., vol. 1, no. 7, pp. 1-11, 2010.
[7] L.M. Varghese, S. Agrawal, D. Sharma, R.P. Mandhan, and R. Mahajan, ”Cost-effective screening and isolation of xylano cellulolytic positive microbes from termite gut and termitarium,” 3 Biotech, vol. 7, no. 2, pp. 108, 2017.
[8] Y.D. Yang, J.Z. Gao, J.H. Wang, R. Hefferman, J. Hanson, K. Paliwal, and Y.Q. Zhou, ”Sixty-five years of the long march in protein secondary structure prediction: the final stretch?,” Briefings in Bioinformatics, vol. 19, no. 3, pp. 482-494, 2018.
[9] S.F. Altschul, W. Gish, W. Miller, E.W. Myers, and D.J. Lipman, ”Basic local alignment search tool,” J. Mol. Biol., vol. 215, no. 3, pp. 403-410, 1990.
[10] F. Madeira, Y.M. Park, J. Lee, N. Buso, T. Gur, N. Madhusoodanan, P. Basutkar, A.R.N. Tivey, S.C. Potter,
R.D. Finn, and R. Lopez, ”The EMBL-EBI search and sequence analysis tools APIs in 2019,”. Nucleic Acids Res., vol. 47, no. W1, pp. W636-W641, 2019.
[11] R.C. Edgar, ”MUSCLE: multiple sequence alignment with high accuracy and high throughput,” Nucleic Acids Res., vol. 32, no. 5, pp. 1792-1797, 2004.
[12] A. Dereeper, V. Guignon, G. Blanc, S. Audic, S. Buffet, F. Chevenet, J.F. Dufayard, S. Guindon, V. Lefort, M. Lescot, J.M. Claverie, and O. Gascuel, ”Phylogeny.fr: robust phylogenetic analysis for the non-specialist,” Nucleic Acids Res., vol. 36, pp. W465-W469, 2008.
[13] A. Waterhouse, M. Bertoni, S. Bienert, G. Studer, G. Tauriello, R. Gumienny, F.T. Heer, T.A.P. de Beer, C. Rempfer, L. Bordoli, R. Lepore, and T. Schwede, ”SWISS-MODEL: homology modelling of protein structures and complexes,”. Nucleic Acids Res., vol. 46, no. W1, pp. W296-W303, 2018.
[14] S. Bienert, A. Waterhouse, T.A.P. de Beer, G. Tauriello, G. Studer, L. Bordoli, and T. Schwede, ”The SWISS-MODEL Repository - new features and functionality,” Nucleic Acids Res., vol. 45, no. D1, pp. D313–D319, 2017.
[15] N. Guex, M.C. Peitsch, and T. Schwede, ”Automated comparative protein structure modeling with SWISS- MODEL and Swiss-PdbViewer: A historical perspective,” Electrophoresis, vol. 30, no. 1, pp. S162-S173, 2009.
[16] P. Benkert, M. Biasini, and T. Schwede, ”Toward the estimation of the absolute quality of individual protein structure models,” Bioinformatics, vol. 27, no. 3, pp. 343-350, 2011.
[17] M. Bertoni, F. Kiefer, M. Biasini, L. Bordoli, and T. Schwede, ”Modeling protein quaternary structure of homo- and hetero-oligomers beyond binary interactions by homology,” Sci Rep., vol. 7, pp. 10480, 2017.
[18] W. Humphrey, A. Dalke, and K. Schulten, ”VMD - Visual molecular dynamics,” J. Mol. Graph., vol. 14, no. 1, pp. 33-38, 1996.
[19] L.A. Plesniak, W.W. Wakarchuk, and L.P. McIntosh, ”Secondary structure and NMR assignments of Bacillus circulans xylanase,” Protein Sci., vol. 5, no. 6, pp. 1118-1135, 1996.
[20] G. Sidhu, S.G. Withers, N.T. Nguyen, L.P. McIntosh, L. Ziser, and G.D. Brayer, ”Sugar ring distortion in the glycosyl-enzyme intermediate of a family G/11 xylanase,” Biochemistry, vol. 38, no. 17, pp. 5346-5354, 1999.
[21] W.W. Wakarchuk, R.L. Campbell, W.L. Sung, J. Davoodi, and M. Yaguchi, ”Mutational and crystallographic analyses of the active site residues of the Bacillus circulans xylanase,” Protein Sci., vol. 3, no. 3, pp. 467-475, 1994.
[22] L. Song, B. Siguier, C. Dumon, S. Bozonnet, and M.J. O’Donohue, ”Engineering better biomass- degrading ability into a GH11 xylanase using a directed evolution strategy,” Biotech. Biofuels, vol. 5, no. 1, pp. 3, 2012.
[23] M.E.S. Soliman, Thesis of: Computational modelling of glycosidase mechanisms: structural and mechanistic aspects. United Kingdom: University of Bath, 2009.