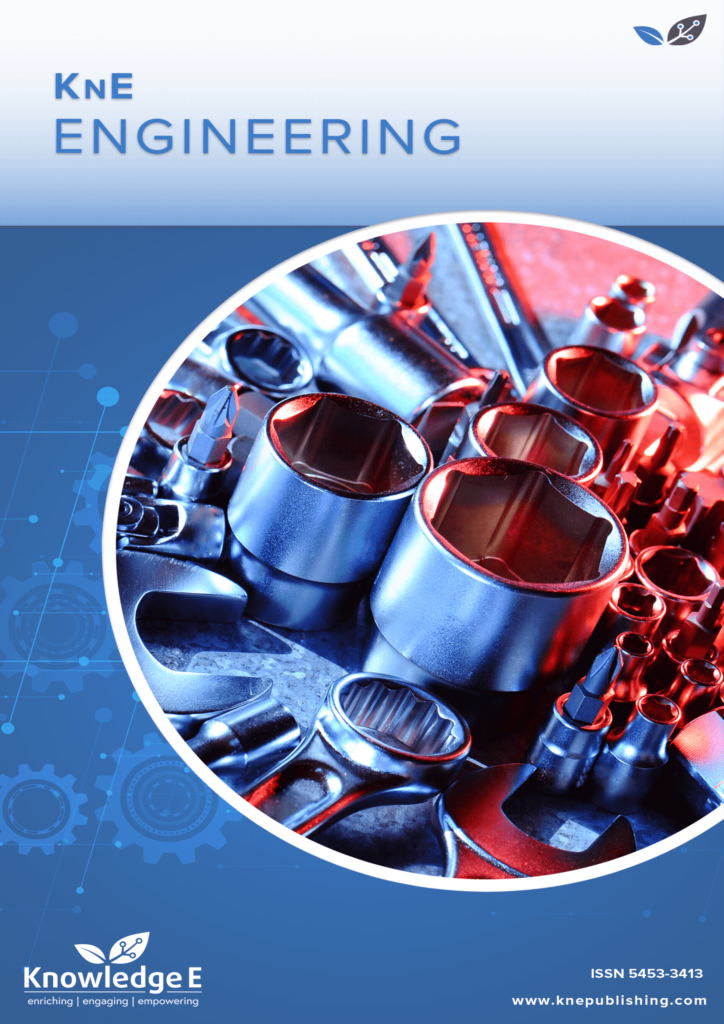
KnE Engineering
ISSN: 2518-6841
The latest conference proceedings on all fields of engineering.
Effect of Group Delay Dispersion on Femtosecond Pulse Self-shortening in Kerr Media: Investigation of Femtosecond Pulse Self-shortening Phenomenon Based on the Transient Regime of Multiple Filamentation in Kerr Media with Different Group Delay Dispersion
Published date: Oct 08 2018
Journal Title: KnE Engineering
Issue title: Breakthrough directions of scientific research at MEPhI: Development prospects within the Strategic Academic Units
Pages: 174–179
Authors:
Abstract:
We present the results of the investigation of the effect of material dispersion on a novel femtosecond pulse self-shortening mechanism based on the transient regime of multiple filamentation in samples with Kerr nonlinearity. This regime occurs at rather high B-integral values for the pulse passing through the sample, so that multiple filamentation in the trailing part causes strong on-axis intensity losses due to diffraction and refraction that allows far-field spatial filtering of the pulse front edge unaffected by the multiple filamentation. Self-shortening of a 72 fs transform-limited pulse to 23 fs, 32 fs and 30 fs was observed in a 1-mm-thick fused silica plate at intensity of 2.9 TW/cm2 , a 3-mm-thick fused silica plate at intensity of 1.1 TW/cm2 and a 5-mm-thick LiF plate at intensity of 2.1 TW/cm2 , respectively, without the use of additional dispersive elements. Experimental results obtained in this article show that the duration of self-shorted pulses increases with increasing group delay dispersion in the material.
Keywords: multiple filamentation, pulse self-shortening
References:
[1] Kobayashi, T., Shirakawa, A., and Fuji, T. (2001). Sub-5-fs transform-limited visible pulse source and its application to real-time spectroscopy. IEEE Journal of Selected Topics in Quantum Electronics, vol. 7, no. 4, pp. 525–538.
[2] Zhou, M. L., Yan, X. Q., Mourou, G., et al. (2016). Proton acceleration by single-cycle laser pulses offers a novel monoenergetic and stable operating regime. Physics of Plasmas, vol. 23, no. 4, p. 043112.
[3] Witte, S., Zinkstok, R. T., Hogervorst, W., et al. (2005). Generation of few-cycle terawatt light pulses using optical parametric chirped pulse amplification. Optics Express, vol. 13, no. 13, pp. 4903–4908.
[4] Rolland, C. and Corkum, P. B. (1988). Compression of high-power optical pulses. JOSA B, vol. 5, no. 3, pp. 641–647.
[5] Nisoli, M., De Silvestri, S., and Svelto, O. (1996). Generation of high energy 10 fs pulses by a new pulse compression technique. Applied Physics Letters, vol. 68, no. 20, pp. 2793–2795.
[6] Stibenz, G., Zhavoronkov, N., and Steinmeyer, G. (2006). Self-compression of millijoule pulses to 7.8 fs duration in a white-light filament. Optics letters, vol. 31, no. 2, pp. 274–276.
[7] Ashihara, S., Nishina, J., Shimura, T., et al. (2002). Soliton compression of femtosecond pulses in quadratic media. JOSA B, vol. 19, no. 10, pp. 2505–2510.
[8] Aristov, A. I., Grudtsyn, Ya. V., Mikheev, L. D., et al. (2012). Spectral broadening and self-compression of negatively chirped visible femtosecond pulses in fused silica. Quantum Electronics, vol. 42, no. 12, pp. 1097–1099.
[9] Alekseev, S. V., Aristov, A. I., Grudtsyn, Ya. V., et al. (2013). Visible-range hybrid femtosecond systems based on a XeF (C–A) amplifier: State of the art and prospects. Quantum Electronics, vol. 43, no. 3, p. 190.
[10] Grudtsyn, Y. V., Zubarev, I. G. E., Koribut, A. V., et al. (2015). Self-phase modulation in a thin fused silica plate upon interaction with a converging beam of down-chirped femtosecond radiation. Quantum Electronics, vol. 45, no. 5, p. 415.
[11] Grudtsyn, Ya. V., Koribut, A. V., Trofimov, V. A., et al. (unpublished). Jellyfish-like mechanism of femtosecond pulse self-shortening in Kerr media. JOSA B.
[12] Bespalov, V. I. and Talanov, V. I. (1966). Filamentary structure of light beams in nonlinear liquids. ZhETF Pisma Redaktsiiu, vol. 3, p. 471.
[13] DeSalvo, R., Said, A. A., Hagan, D. J., et al. (1996). Infrared to ultraviolet measurements of two-photon absorption and n2 in wide bandgap solids. IEEE Journal of Quantum Electronics, vol. 32, no. 8, pp. 1324–1333.
[14] Malitson, I. H. (1965). Interspecimen comparison of the refractive index of fused silica. Josa, vol. 55, no. 10, pp. 1205–1209.
[15] Li, H. H. (1976). Refractive index of alkali halides and its wavelength and temperature derivatives. Journal of physical and chemical reference data, vol. 5, no. 2, pp. 329–528.
[16] Levenson, M. (1974). Feasibility of measuring the nonlinear index of refraction by third-order frequency mixing. IEEE Journal of Quantum Electronics, vol. 10, no. 2, pp. 110–115.
[17] Malitson, I. H. (1963). A redetermination of some optical properties of calcium fluoride. Applied Optics, vol. 2, no. 11, pp. 1103–1107.
[18] Penzkofer, A., Schmailzl, J., and Glas, H. (1982). Four-wave mixing in alkali halide crystals and aqueous solutions. Applied Physics B: Lasers and Optics, vol. 29, no. 1, pp. 37–42.