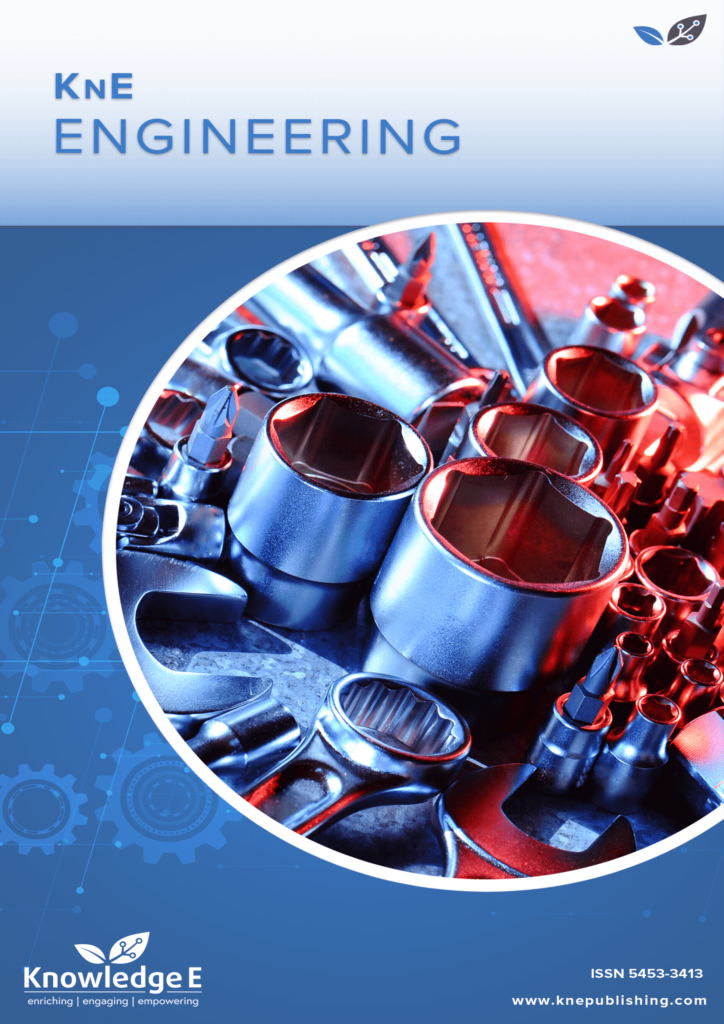
KnE Engineering
ISSN: 2518-6841
The latest conference proceedings on all fields of engineering.
Photoneutrons for Radiation Therapy and Radionuclide Production
Published date: Feb 21 2018
Journal Title: KnE Engineering
Issue title: XIII International Youth Scientific and Practical Conference "FUTURE OF ATOMIC ENERGY - AtomFuture 2017"
Pages: 41-53
Authors:
Abstract:
The possibility of organizing neutron therapy with a photoneutron beam produced by the electron accelerator target, and ensuring the required dose at the tumor at a reasonable exposure time and with minimal impact on patients investigated. Generation of neutrons from the target of electron accelerator takes place in two stages: e- ® γ ® n, and in the selected electron energy range of 20-100 MeV, the bremsstrahlung gamma radiation in many times (~ 3 orders of magnitude) offers more than “useful” neutron yield. This raises the problem of the selective control of the “harmful” for radiotherapy secondary gamma radiation while providing the minimum attenuation of the neutron flux in the output beam. In order to solve the general problem of the formation of a neutron beam with necessary spectral characteristics having sufficient intensity, there has been resolved a number of computational tasks of the selection of the optimal configuration of the output beam unit and its composition. The matter of high importance is to minimize additional irradiation of the patient from the bremsstrahlung (generated by electrons) and secondary gamma radiation (generated by neutrons) from the accelerator target as well as from output unit’s materials. On the other hand, at a generation stage e- ® γ the bremsstrahlung beam could be applied for effective radionuclide production by reactions (γ,n) and (γ,p) due to high leak intensity ~ 1.3·1017 photon/s. By the Mo100(γ,n)99Mo reaction the main diagnostic nuclide 99Tc could be produced sufficiently for the clinical needs. The resulting configuration of the output unit provides the required beam quality for the neutron capture therapy (NCT), which commonly assumed to be the only competitive technology of neutron therapy on the background of the massive invasion of proton therapy and other highly selective techniques that ultimately damage the target sparing the surrounding tissues and organs. For the accessible accelerator (average current 4 mA and electron energy 35 MeV) the flux density of epithermal photoneutrons (they required for NCT) in the beam at the output is of the order of magnitude or more higher than the typical yield from existing and planned reactors' beams. The proposed scheme of generation and extraction of photoneutrons for NCT has a number of strong advantages over traditional techniques: a) the applying of electron accelerators for neutron production is much safer and cheaper than conventional reactor beams in use; b) accelerator with the target, the beam output unit with the necessary equipment can be placed on the territory of the clinic without any problems of radiation safety; c) the proposed target – liquid gallium, which also serves as a cooler, is an “environmentally friendly” material due to low activation which rapidly (in ~ 4 days) falls to the background level.
References:
[1] Kurachenko Yu.A., Voznesensky N.K., Goverdovsky A.A., Rachkov V.I. Novyi intensivnyi istochnik nejtronov dlya medicinskih prilozhenij [New intensive neutron source for medical application]. Medicinskaya fizika. 2012, no. 2 (38), pp. 29–38. (in Russian).
[2] Kurachenko Yu.A. Fotonejtrony dlya nejtronozahvatnoj terapii [Photoneutrons for neutron capture therapy]. Izvestiya vuzov. Yadernaya energetika. 2014, no. 4, pp. 41 – 51. (in Russian).
[3] Kurachenko Yu.A., Zabaryansky Yu.G., Onischuk H.A. Optimizatsiya misheni dlya proizvodstva fotonejtronov [Optimization of the target for photoneutron production]. Izvestiya vuzov. Yadernaya energetika. 2016, No. 3, pp. 150 – 162. (in Russian).
[4] Kurachenko Yu.A., Zabaryansky Yu.G., Onischuk H.A. Primenenie fotonejtronov dlya luchevoj terapii [Photoneutrons application for radiation therapy]. Medicinskaya radiologiya i radiatsionnaya bezopasnost’. 2017, v. 62, No. 3, pp. 33 – 42. (in Russian).
[5] High Power Linacs for Isotope Production. MEVEX: The accelerator technology company. Internet resource http://www.mevex.com/Brochures/Brochure_High_ Energy.pdf.
[6] MCNP – A General Monte Carlo N-Particle Transport Code, Version 5. Volume I: Overview and Theory. Authors: X-5 Monte Carlo Team //LA-UR-03-1987.April 24, 2003.
[7] STAR-CD®. Internet resource CD-adapco Engineering Simulation Software - CAE and CFD Software.
[8] The Basics of Boron Neutron Capture Therapy. Internet resource http://web.mit. edu/nrl/www/bnct/facilities/facilities.html
[9] MIT BNCT Facilities. Fission Converter Beam (FCB). Internet resource http://web. mit.edu/nrl/www/bnct/facilities/MITBNCTFacilities.htm
[10] Reattore TAPIRO: ENEA Internal Document, DISP/TAP/85-1, 1985. In: Design of neutron beams for boron neutron capture therapy in a fast reactor//Current status of neutron capture therapy, IAEA-TECDOC-1223, 2001.
[11] G. Rosi et al. ROLE OF THE TAPIRO FAST RESEARCH REACTOR IN NEUTRON CAPTURE THERAPY IN ITALY. Calculations and measurements // IAEA-CN-100/97. In: Research Reactor Utilization, Safety, Decommissioning, Fuel and Waste Management Proceedings of an international conference 10–14 November 2003 Santiago, Chile. pp. 325-338.
[12] Carta M., Palomba M.. TRIGA RC-1 and TAPIRO ENEA Research Reactors. Internet resource: https://www.iaea.org/OurWork/ST/NE/NEFW/Technical-Areas/RRS/ documents/TM_Innovation/Carta_ENEA.pdf.
[13] General information and technical data of TAPIRO research reactor. Internet resource http://www.enea.it/en/research-development/documents/nuclearfission/tapiro-eng-pdf.
[14] Nuclear Research Reactor: TAPIRO. Internet resource http://old.enea.it/com/ ingl/New_ingl/research/energy/nucleare_fission/pdf/TAPIRO-ENG.pdf.
[15] Kurachenko Yu.A., Kazanskij Yu.A., Levchenko A.B., Matusevich Eu.S. Vyvod nejtronnyh puchkov i zaschita meditcinskogo reaktora MARS [Neutron beam’s removing and protection for the medical MARS reactor]. Izvestiya vuzov. Yadernaya energetika. 2006, no. 4, pp. 36–48. (in Russian).
[16] Kurachenko Yu.A., Moiseenko D.N. MARS i TAPIRO: reaktory maloj moschnosti dlya nejtrono-zahvatnoj terapii [MARS & TAPIRO: small-capacity reactors for neutron capture therapy]. Izvestiya vuzov. Yadernaya energetika. 2010, no. 1, pp. 153–163. (in Russian).
[17] Kurachenko Yu.A., Kazanskij Yu.A., Matusevich Eu.S. Kriterii kachestva nejtronnyh puchkov dlya luchevoj terapii [Neutron beams’ quality criteria for radiation therapy]. Izvestiya vuzov. Yadernaya energetika. 2008, no. 1, pp. 139–149. (in Russian).
[18] Kurachenko Yu.A. Reaktornye puchki dlya luchevoj terapii: kriterii kachestva i raschyotnye tekhnologii [Reactor beams for the radiation therapy: quality criteria and computation technologies]. Medicinskaya fizika. 2008, no. 2 (38), pp. 20–28. (in Russian).
[19] Kurachenko Yu. A. Reaktornye puchki dlya luchevoj terapii. Raschyotnye modeli i vychislitel’nye tekhnologii [Reactor beams for radiation therapy. Calculation models and computation technologies]. Palmarium Academic Publishing, OmniScriptum GmbH&Co. RG, Saarbrücken, Deutschland. (ISBN: 978-3-8473-9842-4) 2013. 372 p.
(in Russian).