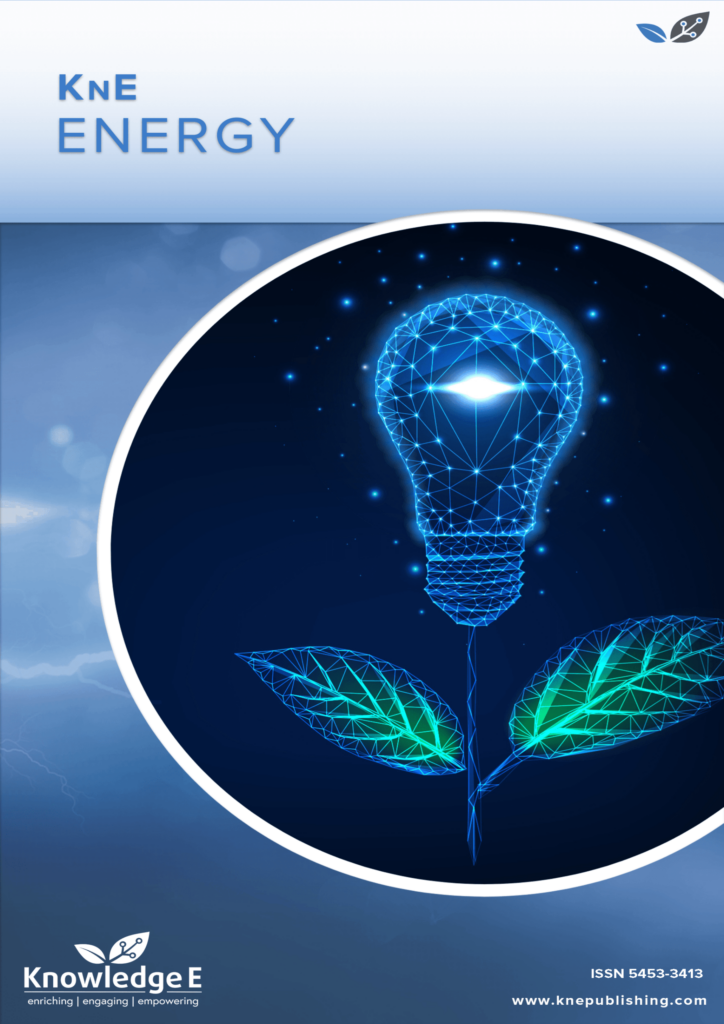
KnE Energy
ISSN: 2413-5453
The latest conference proceedings on energy science, applications and resources
The Effect of Quantum Dot Shell Structure on Fluorescence Quenching By Acridine Ligand
Published date:Apr 17 2018
Journal Title: KnE Energy
Issue title: The 2nd International Symposium "Physics, Engineering and Technologies for Biomedicine"
Pages:194–201
Authors:
Abstract:
The current strategy for the development of advanced methods of tumor treatment focuses on targeted drug delivery to tumor cells. Quantum dot (QD) - semiconductor fluorescent nanocrystal, conjugated with a pharmacological ligand, such as acridine, ensures real-time tracking of the delivery process of the active substance. However, the problem of QD fluorescence quenching caused by charge transfer can arise in the case when acridine is bound to the QD. We found that QD shell structure has a defining role on photoinduced electron transfer from QD on acridine ligand which leads to quenching of QD photoluminescence. We have found that multishell CdSe/ZnS/CdS/ZnS QD structure provides minimal reduction of photoluminescence quantum yield at minimal shell thickness compared to classical thin ZnS or “giant” shells. Thus, CdSe/ZnS/CdS/ZnS core/multishell QD could be an optimal choice for engineering of small-sized acridine-based fluorescent labels for tumor diagnosis and treatment systems.
Keywords: Quantum dot, photoluminescence quenching, DNA ligand, acridine derivative.
References:
[1] Nagy A. et al. Comprehensive analysis of the effects of CdSe quantum dot size, surface charge, and functionalization on primary human lung cells. // ACS nano. 2012. Vol. 6, № 6. P. 4748–4762.
[2] Shi J. et al. Nanotechnology in Drug Delivery and Tissue Engineering: From Discovery to Applications // Nano Letters. American Chemical Society, 2010. Vol. 10, № 9. P. 3223–3230.
[3] Sukhanova A. et al. Oriented conjugates of single-domain antibodies and quantum dots: toward a new generation of ultrasmall diagnostic nanoprobes. // Nanomedicine: nanotechnology, biology, and medicine. 2012. Vol. 8, № 4. P. 516– 525.
[4] Bilan R. et al. Quantum Dot Surface Chemistry and Functionalization for Cell Targeting and Imaging // Bioconjugate Chemistry. 2015. Vol. 26, № 4. P. 609–624.
[5] Linkov P. et al. Multifunctional Nanoprobes for Cancer Cell Targeting, Imaging and Anticancer Drug Delivery // Physics Procedia. 2015. Vol. 73. P. 216–220.
[6] Samokhvalov P., Artemyev M., Nabiev I. Basic principles and current trends in colloidal synthesis of highly luminescent semiconductor nanocrystals. // Chemistry - Eur. J. 2013. Vol. 19, № 5. P. 1534–1546.
[7] Dovzhenko D. et al. Enhancement of Spontaneous Emission from CdSe/CdS/ZnS Quantum Dots at the Edge of the Photonic Band Gap in a Porous Silicon Bragg Mirror// Physics Procedia. 2015. Vol. 73. P. 126–130.
[8] Linkov P. et al. Ultrasmall Quantum Dots: A Tool for in Vitro and in Vivo Fluorescence Imaging // Journal of Physics: Conference Series. 2017. Vol. 784. P. 12033.
[9] Zhao J.-Y. et al. Ultrasmall Magnetically Engineered Ag 2 Se Quantum Dots for Instant Efficient Labeling and Whole-Body High-Resolution Multimodal Real-Time Tracking of Cell-Derived Microvesicles // Journal of the American Chemical Society. 2016. Vol. 138, № 6. P. 1893–1903.
[10] Wegner K.D., Hildebrandt N. Quantum dots: bright and versatile in vitro and in vivo fluorescence imaging biosensors // Chem. Soc. Rev. 2015. Vol. 44, № 14.
[11] Sparapani S. et al. Rational design of acridine-based ligands with selectivity for human telomeric quadruplexes // Journal of the American Chemical Society. 2010. Vol. 132, № 35. P. 12263–12272.
[12] Laronze-Cochard M. et al. Synthesis and biological evaluation of novel 4,5- bis(dialkylaminoalkyl)-substituted acridines as potent telomeric G-quadruplex ligands // European Journal of Medicinal Chemistry. 2009. Vol. 44, № 10. P. 3880– 3888.
[13] Burger A.M. The G-Quadruplex-Interactive Molecule BRACO-19 Inhibits Tumor Growth, Consistent with Telomere Targeting and Interference with Telomerase Function // Cancer Research. 2005. Vol. 65, № 4. P. 1489–1496.
[14] Müller S. et al. Small-molecule-mediated G-quadruplex isolation from human cells // Nature Chemistry. Nature Research, 2010. Vol. 2, № 12. P. 1095–1098.
[15] Artese A. et al. Toward the design of new DNA G-quadruplex ligands through rational analysis of polymorphism and binding data // European Journal of Medicinal Chemistry. 2013. Vol. 68. P. 139–149.
[16] Cho A.-N. et al. Acridine-based novel hole transporting material for high efficiency perovskite solar cells // J. Mater. Chem. A. The Royal Society of Chemistry, 2017. Vol. 5, № 16. P. 7603–7611.
[17] Krzymiński K. et al. 1H and 13C NMR spectra, structure and physicochemical features of phenyl acridine-9-carboxylates and 10-methyl-9-(phenoxycarbonyl)acridinium trifluoromethanesulphonates – alkyl substituted in the phenyl fragment // Spectrochimica Acta Part A: Molecular and Biomolecular Spectroscopy. 2011. Vol. 78, № 1. P. 401–409.
[18] Jasieniak J., Califano M., Watkins S.E. Size-Dependent Valence and Conduction Band- Edge Energies of Semiconductor Nanocrystals // ACS Nano. 2011. Vol. 5, № 7. P. 5888–5902.
[19] Islam A. et al. Blue light-emitting devices based on 1,8-acridinedione derivatives // Synthetic Metals. 2003. Vol. 139, № 2. P. 347–353.
[20] Liu I.-S. et al. Enhancing photoluminescence quenching and photoelectric properties of CdSe quantum dots with hole accepting ligands // Journal of Materials Chemistry. 2008. Vol. 18, № 6. P. 675.
[21] Krivenkov V.A. et al. Surface ligands affect photoinduced modulation of the quantum dots optical performance // SPIE Proceedings. 2014. Vol. 9126. P. 91263N.
[22] Bang J. et al. Photoswitchable quantum dots by controlling the photoinduced electron transfers // Chemical Communications. 2012. Vol. 48, № 73. P. 9174.
[23] Linkov P. et al. Ultrasmall Quantum Dots for Fluorescent Bioimaging in vivo and in vitro // Optics and Spectroscopy. 2017. Vol. 122, № 1. P. 8–11.
[24] Dubal D.P., Holze R. A successive ionic layer adsorption and reaction (SILAR) method to induce Mn3O4 nanospots on CNTs for supercapacitors // New Journal of Chemistry. 2013. Vol. 37, № 2. P. 403.
[25] Samokhvalov P. et al. Photoluminescence quantum yield of CdSe-ZnS/CdS/ZnS core-multishell quantum dots approaches 100
[26] Chen Y. et al. “Giant” multishell CdSe nanocrystal quantum dots with suppressed blinking. // Journal of the American Chemical Society. 2008. Vol. 130, № 15. P. 5026– 5027.
[27] Razgoniaeva N. et al. One-dimensional carrier confinement in “ giant ” CdS / CdSe excitonic nanoshells. 2017.
[28] Linkov P. et al. Comparative advantages and limitations of the basic metrology methods applied to the characterization of nanomaterials. // Nanoscale. 2013. Vol. 5, № 19. P. 8781–8798.
[29] Jasieniak J. et al. Re-examination of the Size-Dependent Absorption Properties of CdSe Quantum Dots // The Journal of Physical Chemistry C. American Chemical Society, 2009. Vol. 113, № 45. P. 19468–19474.
[30] Linkov P. et al. High Quantum Yield CdSe/ZnS/CdS/ZnS Multishell Quantum Dots for Biosensing and Optoelectronic Applications // Materials Today: Proceedings. 2016. Vol. 3, № 2. P. 104–108.