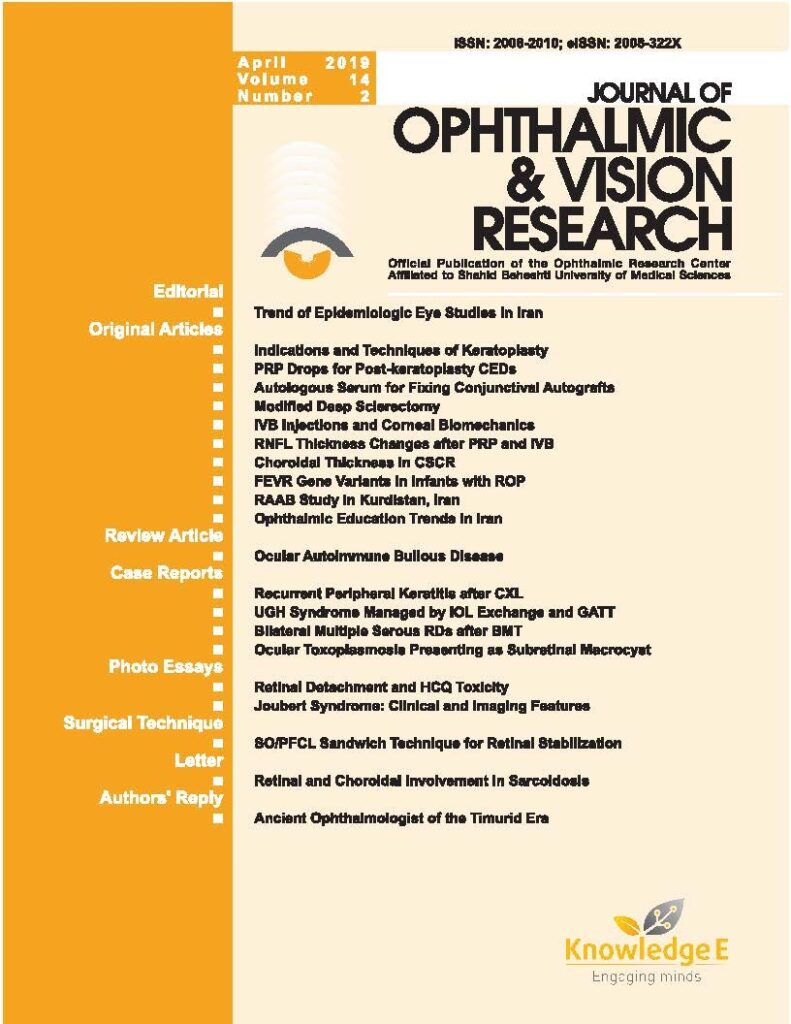
Journal of Ophthalmic and Vision Research
ISSN: 2008-322X
The latest research in clinical ophthalmology and vision science
Modification of a Selective NTRK2 Agonist and Confirmation of Activity in a Glaucoma-on-a-Chip Model
Published date:Mar 10 2024
Journal Title: Journal of Ophthalmic and Vision Research
Issue title: Jan–Mar 2024, Volume 19, Issue 1
Pages:58 - 70
Authors:
Abstract:
Purpose: RNYK is a selective agonist of the neurotrophic tyrosine kinase receptor type 2 (NTRK2) which has been screened from a phage-displayed peptide library. Its sequence is SGVYKVAYDWQH, similar to a native NTRK2 ligand, that is, brain-derived neurotrophic factor (BDNF). The current study was performed to recognize and confirm critical residues for RNYK activity in a glaucoma-on-a-chip model.
Methods: We designed a modified RNYK (mRNYK) peptide based on hotspots of the RNYK sequence identified by alanine scanning. The critical residues consisted of tyrosine, valine, aspartic acid, and tryptophan (YVDW); however, lysine and glutamine were also maintained in the final sequence (YKVDWQ) for forming amide bonds and peptide dimerization. The affinity of mRNYK binding was confirmed by testing against NTRK2 receptors on the surface of ATRA-treated SH-SY5Y cells. The neuroprotective effect of mRNYK was also evaluated in cell culture after elevated pressure insult in a glaucoma-on-a-chip model.
Results: The primary amine on the lysine side-chain from one sequence (YKVDWQ) reacted with a γ[1]carboxamide group of glutamine from the other sequence, forming dimeric mRNYK. In silico, molecular dynamic simulations of the mRNYK–NTRK2 complex showed more stable and stronger interactions as compared to the RNYK–NTRK2 complex. In vitro, mRNYK demonstrated a neuroprotective effect on SH-SY5Y cells under normal and elevated pressure comparable to RNYK. The 50% effective concentration (logEC50) for mRNYK was 0.7009, which was better than RNYK with a logEC50 of 0.8318.
Conclusion: The modified peptide studied herein showed improved stability over the original peptide (RNYK) and demonstrated potential for use as a BDNF agonist with neuroprotective properties for treatment of neurodegenerative disorders such as glaucoma.
Keywords: Agonist, Brain-derived Neurotrophic Factor, Neuroprotection, Neurotrophic Tyrosine Receptor Kinase
References:
1. Soppet D, Escandon E, Maragos J, Middlemas DS, Raid SW, Blair J, et al. The neurotrophic factors brain-derived neurotrophic factor and neurotrophin-3 are ligands for the trkB tyrosine kinase receptor. Cell 1991;65:895–903.
2. Carter BD, Kaltschmidt C, Kaltschmidt B, Offenhäuser N, Böhm-Matthaei R, Baeuerle PA, et al. Selective activation of NF-κB by nerve growth factor through the neurotrophin receptor p75. Science 1996;272:542–545.
3. Banfield MJ, Naylor RL, Robertson AG, Allen SJ, Dawbarn D, Brady RL. Specificity in Trk receptor: Neurotrophin interactions: The crystal structure of TrkB-d5 in complex with neurotrophin-4/5. Structure 2001;9:1191–1199.
4. Kaplan DR, Miller FD. Neurotrophin signal transduction in the nervous system. Curr Opin Neurobiol 2000;10:381– 391.
5. Barker PA. p75NTR is positively promiscuous: Novel partners and new insights. Neuron 2004;42:529–533.
6. Nafian F, Rasaee MJ, Yazdani S, Soheili ZS. Discovery of novel peptidomimetics for brain-derived neurotrophic factor using phage display technology. Iran J Pharm Res 2018;14:9–20.
7. Nafian F, Rasaee MJ, Yazdani S, Daftarian N, Soheili ZS, Kamali Doust Azad B. Peptide selected by phage display increases survival of SH-SY5Y neurons comparable to brain-derived neurotrophic factor. J Cell Biochem 2019;120:7612–7622.
8. Morris GM, Huey R, Lindstrom W, Sanner MF, Belew RK, Goodsell DS, et al. AutoDock4 and AutoDockTools4: Automated docking with selective receptor flexibility. J Comput Chem 2009;30:2785–2791.
9. Ultsch MH, Wiesmann C, Simmons LC, Henrich J, Yang M, Reilly D, et al. Crystal structures of the neurotrophinbinding domain of TrkA, TrkB, and TrkC. J Mol Biol 1999;290:149–159.
10. Kastin A. Handbook of biologically active peptides. Academic Press; 2013.
11. Lamiable A, Thévenet P, Rey J, Vavrusa M, Derreumaux P, Tufféry P. PEP-FOLD3: Faster de novo structure prediction for linear peptides in solution and in complex. Nucleic Acids Res 2016;44:W449–W454.
12. Souza FR, Souza LMP, Pimentel AS. Recent open issues in coarse-grained force fields. J Chem Inf Model 2020;60:5881–5884.
13. Eswar N, Eramian D, Webb B, Shen M-Y, Sali A. Protein structure modeling with MODELLER. Structural Proteomics. Springer; 2008;1:145159.
14. Xu D, Zhang Y. Improving the physical realism and structural accuracy of protein models by a two-step atomic-level energy minimization. Biophys J 2011;101:2525–2534.
15. Robertson MJ, Tirado-Rives J, Jorgensen WL. Improved peptide and protein torsional energetics with the OPLS-AA force field. J Chem Theory Comput 2015;11:3499–3509.
16. Guo L, Lin W, Zhang Y, Wang J. A systematic analysis revealed the potential gene regulatory processes of ATRA-triggered neuroblastoma differentiation and identified a novel RA response sequence in the NTRK2 gene. Biomed Res Int 2020;2020:6734048.
17. Ranjan S, Sood R, Dudas J, Glueckert R, Schrott-Fischer A, Roy S, et al. Peptide-mediated targeting of liposomes to TrkB receptor-expressing cells. Int J Nanomedicine 2012;7:3475–3485.
18. Do H, Park H-J, Sohn E-H, Kim B-O, Um SH, Kwak J-H, et al. Ethanol induces cell cycle arrest and triggers apoptosis via Sp1-dependent p75NTR expression in human neuroblastoma cells. Cell Biol Toxicol 2013;29:365–380.
19. Nafian F, Kamali Doust Azad B, Yazdani S, Rasaee MJ, Daftarian N. A lab-on-a-chip model of glaucoma. Brain Behav 2020;10:e01799.
20. Proniewicz E, Burnat G, Domin H, Małuch I, Makowska M, Prahl A. Application of alanine scanning to determination of amino acids essential for peptide adsorption at the solid/solution interface and binding to the receptor: Surface-enhanced raman/infrared spectroscopy versus bioactivity assays. J Med Chem 2021;64:8410–8422.
21. Liu X, Peng L, Zhou Y, Zhang Y, Zhang JZ. Computational alanine scanning with interaction entropy for protein–ligand binding free energies. J Chem Theory Comput 2018;14:1772–1780.
22. Kortemme T, Baker D. Computational design of protein–protein interactions. Curr Opin Chem Biol 2004;8:91–97.
23. Wood CW, Ibarra AA, Bartlett GJ, Wilson AJ, Woolfson DN, Sessions RB. BAlaS: Fast, interactive and accessible computational alanine-scanning using BudeAlaScan. Bioinformatics 2020;36:2917–2919.
24. McIntosh-Smith S, Price J, Sessions RB, Ibarra AA. High performance in silico virtual drug screening on many-core processors. Int J High Perform Comput Appl 2015;29:119–134.
25. Korecka JA, van Kesteren RE, Blaas E, Spitzer SO, Kamstra JH, Smit AB, et al. Phenotypic characterization of retinoic acid differentiated SH-SY5Y cells by transcriptional profiling. PloS One 2013;8:e63862.
26. Kovalevich J, Santerre M, Langford D. Considerations for the use of SH-SY5Y neuroblastoma cells in neurobiology. Methods Mol Biol 2021;2311:9–23.
27. Encinas M, Iglesias M, Liu Y, Wang H, Muhaisen A, Cena V, et al. Sequential treatment of SH-SY5Y cells with retinoic acid and brain-derived neurotrophic factor gives rise to fully differentiated, neurotrophic factor-dependent, human neuron-like cells. J Neurochem 2000;75:991–1003.
28. Encinas M, Iglesias M, Llecha N, Comella J. Extracellular regulated kinases and phosphatidylinositol 3-kinase are involved in brain-derived neurotrophic factor-mediated survival and neuritogenesis of the neuroblastoma cell line SH-SY5Y. J Neurochem 1999;73:1409–1421.
29. Park HJ, Do H, Sohn EH, Rhee DK, Pyo S. Ethanol induced cell cycle arrest and apoptosis is mediated by sp1- dependent expression of p75NTR in neuroblastoma cells. Wiley Online Library; 2011.
30. Kenneth Morrow J, Zhang S. Computational prediction of protein hot spot residues. Curr Pharma Design 2012;18:1255–1265.
31. Bogan AA, Thorn KS. Anatomy of hot spots in protein interfaces. J Mol Biol 1998;280:1–9.
32. O’Leary P, Hughes R. Structure-activity relationships of conformationally constrained peptide analogues of loop 2 of brain-derived neurotrophic factor. J Neurochem 1998;70:1712–1721.
33. O’Leary PD, Hughes RA. Design of potent peptide mimetics of brain-derived neurotrophic factor. J Biol Chem 2003;278:25738–25744.
34. Fletcher JM, Hughes RA. Novel monocyclic and bicyclic loop mimetics of brain-derived neurotrophic factor. J Pept Sci 2006;12:515–524.
35. Aires ID, Ambrósio AF, Santiago AR. Modeling human glaucoma: Lessons from the in vitro models. Ophthal Res 2017;57:77–86.
36. Liu B, Ma X, Guo D, Guo Y, Chen N, Bi H. Neuroprotective effect of alpha-lipoic acid on hydrostatic pressure-induced damage of retinal ganglion cells in vitro. Neurosci Lett 2012;526:24–28.
37. Zhang S-H, Gao F-J, Sun Z-M, Xu P, Chen J-Y, Sun X-H, et al. High pressure-induced mtDNA alterations in retinal ganglion cells and subsequent apoptosis. Front Cell Neurosci 2016;10:254.
38. Miao H, Crabb AW, Hernandez MR, Lukas TJ. Modulation of factors affecting optic nerve head astrocyte migration. Invest Ophthalmol Vis Sci 2010;51:4096–4103.
39. Mandal A, Shahidullah M, Delamere NA. Hydrostatic pressure–induced release of stored calcium in cultured rat optic nerve head astrocytes. Invest Ophthalmol Vis Sci 2010;51:3129–3138.
40. Yu J, Chen C, Wang J, Cheng Y, Wu Q, Zhong Y, et al. In vitro effect of adenosine on the mRNA expression of Kir 2.1 and Kir 4.1 channels in rat retinal Müller cells at elevated hydrostatic pressure. Exp Ther Med 2012;3:617–620.
41. Yu J, Zhong Y, Shen X, Cheng Y, Qi J, Wang J. In vitro effect of adenosine A2A receptor antagonist SCH 442416 on the expression of glutamine synthetase and glutamate aspartate transporter in rat retinal Müller cells at elevated hydrostatic pressure. Oncol Rep 2012;27:748–752.
42. Madeira MH, Elvas F, Boia R, Gonçalves FQ, Cunha RA, Ambrósio AF, et al. Adenosine A 2A R blockade prevents neuroinflammation-induced death of retinal ganglion cells caused by elevated pressure. J Neuroinflammation 2015;12:115128.
43. Yu J, Zhong Y, Cheng Y, Shen X, Wang J, Wei Y. Effect of high hydrostatic pressure on the expression of glutamine synthetase in rat retinal Müller cells cultured in vitro. Exp Ther Med 2011;2:513–516.
44. Tök L, Nazıroğlu M, Uğuz AC, Tök Ö. Elevated hydrostatic pressures induce apoptosis and oxidative stress through mitochondrial membrane depolarization in PC12 neuronal cells: A cell culture model of glaucoma. J Recept Signal Transduction 2014;34:410–416.
45. Osborne A, Aldarwesh A, Rhodes JD, Broadway DC, Everitt C, Sanderson J. Hydrostatic pressure does not cause detectable changes in survival of human retinal ganglion cells. PloS One 2015;10:e0115591.
46. Lee JK, Lu S, Madhukar A. Real-time dynamics of Ca2+, caspase-3/7, and morphological changes in retinal ganglion cell apoptosis under elevated pressure. PloS One 2010;5:e13437.
47. Ohnishi T, Sakamoto K, Asami-Odaka A, Nakamura K, Shimizu A, Ito T, et al. Generation of a novel artificial TrkB agonist, BM17d99, using T7 phage-displayed random peptide libraries. Biochem Biophys Res Commun 2017;483:101–106.